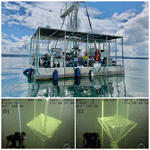
Hipercorig – an innovative hydraulic coring system recovering over 60 m long sediment cores from deep perialpine lakes
Ulli Raschke
Flavio S. Anselmetti
Michael Strasser
Volker Wittig
Martin Wessels
Sebastian Schaller
Stefano C. Fabbri
Richard Niederreiter
Antje Schwalb
The record of past environmental conditions and changes archived in lacustrine sediments serves as an important element in paleoenvironmental and climate research. A main barrier in accessing these archives is the undisturbed recovery of long cores from deep lakes. In this study, we have developed and tested a new, environmentally friendly coring tool and modular barge, centered around a down-the-hole hydraulic hammering of an advanced piston coring system, called the Hipercorig. Test beds for the evaluation of the performance of the system were two periglacial lakes, Mondsee and Constance, located on the northern edge of the Alpine chain. These lakes are notoriously difficult to sample beyond ∼ 10 m sediment depths due to dense glacial deposits obstructing deeper coring. Both lakes resemble many global lake systems with hard and coarse layers at depth, so the gained experience using this novel technology can be applied to other lacustrine or even marine basins.
These two experimental drilling projects resulted in up to 63 m coring depth and successful coring operations in up to 204 m water depth, providing high-quality, continuous cores with 87 % recovery. Initial core description and scanning of the 63 m long core from Mondsee and two 20 and 24 m long cores from Lake Constance provided novel insights beyond the onset of deglaciation of the northern Alpine foreland dating back to ∼ 18 400 cal BP.
- Article
(36975 KB) - Full-text XML
- BibTeX
- EndNote
Many lakes have been identified as outstanding recorders of local, regional, and sometimes even global climate and environment in high resolution and with long-lasting continuity over several glacial–interglacial cycles. These archives cover major parts of the Quaternary (Litt and Anselmetti, 2014; Johnson et., 2016; Wagner et al., 2019; Melles et al., 2012) and beyond (Brigham-Grette et al., 2013). However, the identification of lacustrine strata bearing highly resolved time series requires studies that show high-quality proxy data and, at the same time, radiometric dating possibilities. Such investigations are usually performed on cores (∼ 10–15 m long) that can be recovered using inexpensive gravity or piston coring devices. In order to access sediment archives with a long geological history, as a first step, hydro-acoustic data (bathymetric and seismic data) need to be acquired to elucidate if such promising strata continue to greater depth. The second step is to plan and conduct coring to depths of at least several decameters. This requires, in most cases, drilling operations conducted with the help of commercial contractors to ensure the achievement of sediment depths beyond 20 m. Because costs for coring deep, continuous, and undisturbed strata from large lakes require budgets in the range of USD 1 million, well-developed project proposals often involve complex, lengthy international funding efforts before they can be realized.
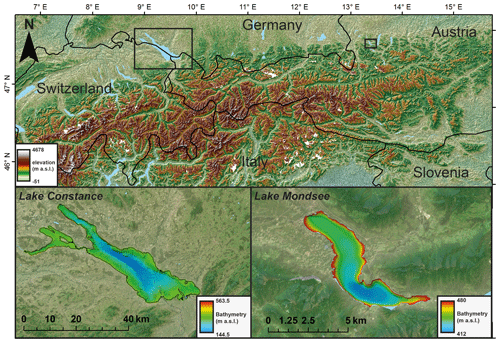
Figure 1Digital elevation model of the Alpine chain in central Europe based on Shuttle Radar Topography Mission (SRTM) 1 arcsec imagery (30 m; USGS Explorer) with borders as solid black lines and all major lakes in blue. Insets show lakes Constance (Wessels et al., 2015; bathymetry and offshore lidar) and Mondsee, with bathymetric information (data from Mondsee bathymetry courtesy of Immo Trinks, Ludwig Boltzmann Institute for Archaeological Prospection and Virtual Archaeology, Vienna) superimposed using Universal Transverse Mercator (UTM) coordinates. The inset maps combine elevation data with topographic maps (orthographic aerial and satellite imagery from Bing Maps, © Microsoft Corporation).
Our approach to addressing the lack of easily available coring techniques to recover up to 100 m long sediment cores was twofold, as follows:
-
Design, construct and field-test a novel, high-quality soft sediment coring system that allows penetration beyond today's hand-driven piston corers, while remaining, economically, well below industry coring operations.
-
Test the new coring system on two lakes in which sediments are known to resist standard coring attempts that are hitherto unexplored but bear high scientific potential.
We have chosen Mondsee in Austria and the transboundary Lake Constance in Austria, Germany, and Switzerland (Fig. 1) as test beds because previous attempts to core deeper than 14 m in both basins failed. At the same time, the previously recovered Holocene clay-rich lake deposits include a wealth of data on the environmental, climate, and hydrologic evolution of these perialpine basins (see e.g., Wessels et al., 1999; Schwalb et al., 2013; Daxer et al., 2018 and references therein; Blattmann et al., 2020).
This study provides insights into the technique of the new coring system named Hipercorig (derived from “high percussion coring rig”) and its deployment on the test lakes. The core material recovered from 63 m depth in Mondsee and at 204 m water depth in Lake Constance is described, and the first analytical results are discussed.
Hipercorig consists of a highly mobile modular barge system, a coring rig, a hydraulic piston coring device, steel casings, lake bottom ground plate and winches, ropes, and other auxiliary equipment (Fig. 2) that can all be packed and shipped in four 20 ft (6 m) standard shipping containers. The platform is 6.3 × 8 m in size, comprising 14 floating tanks of aluminum of 2, 3, or 4 m × 1 × 1 m dimension. A moon pool (2 × 2 m) in the center ensures centralized lowering of all underwater equipment via the drill rig. The barge is self-propelled by two outboard engines up to a speed of 9 km h−1 and accompanied by two outboard-engine-driven rubber boats, also stored in the containers. The barge has a draft of 30 to 40 cm, and its freeboard can be adjusted using four blow ballast tanks, depending on needs for speedier taxi or wave action dampening on-site.
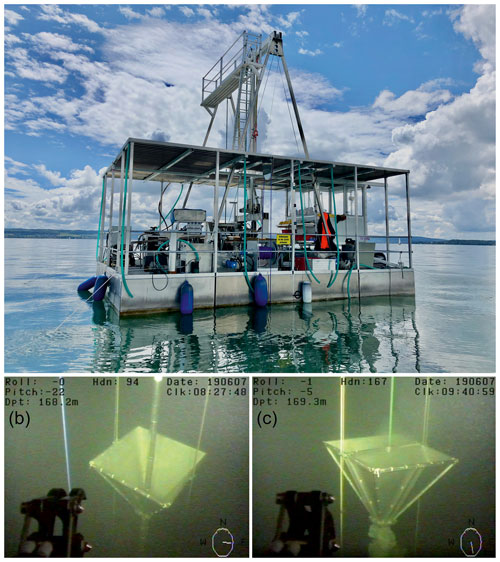
Figure 2Hipercorig on Lake Constance during coring operations in 2019 (a). Video stills from research vessel (R/V) Kormoran remotely operated vehicle (ROV) showing the entry of coring assembly in the upper reentry cone (b). The reentry cone with hydraulic hose (green) after the coring assembly was lowered into the hole (c).
At coring position, the four Bruce-type anchors are each set on 700 m of Kevlar rope and placed outwards from the barge to keep station. Precise stationing and readjustment perpendicular to the drill site are achieved with four hydraulic anchor winches. Coring operations are prepared by lowering a 4 m high ground plate through the moon pool with four winged basal plates to the bottom of the lake (Fig. 3). It is applied to ensure a vertical angle of the coring equipment to the lake bottom. Entry for the casing pipe is provided through a 1.3 × 1.3 m reentry cone mounted on top of the base plate.
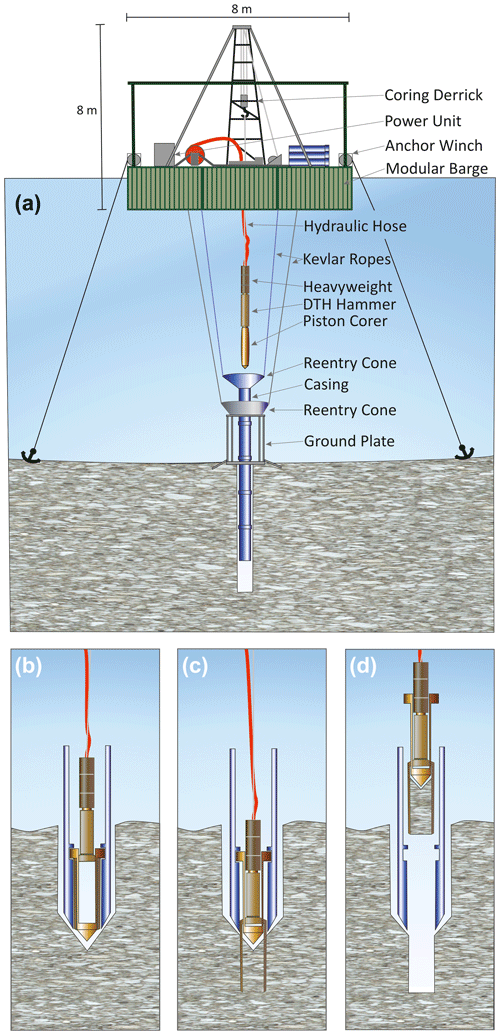
Figure 3Scheme of Hipercorig key elements, with the ground plate set and coring assembly lowered half-way (a). Illustration of coring operations with the coring assembly placed in the hole (b, the corer hammered into sediment (c), and the extraction of coring assembly, including the new core, to the platform while the casing remains in place (d).
Hoisting and mounting of the ground plate and all underwater equipment is done using a 7 m high coring rig. A 46 kW gasoline engine drives, through a hydraulic aggregate, all winches and motors, including the four anchor winches and winches for the ground plate, casing, coring unit, hydraulic hose, and data cable, with biodegradable oil. In the following step, 2 m long pipe sections, together forming an up to 100 m long casing, are connected with a pipe spinner rotary unit and are finally topped by an upper reentry cone. This casing pipe setup is lowered to the ground plate through the reentry cone in the right position.
Coring preparation starts on the drilling platform by assembling the core barrel unit, hammer unit, and heavyweight pipes, i.e., the innovative centerpiece of Hipercorig. It is a combination of a hydraulic down-the-hole (DTH) hammer with a proven piston coring system. The principles of the piston corer are described, e.g., in Gallmetzer et al. (2016) and references therein. The water-driven DTH hammer (e.g., Wassara W 50 hammer, with up to 170 bar and about 100 L water min−1 consumption), developed originally for deep drilling or mining, is connected to the piston corer with a special adaptor. The key advantage of such a DTH hammer is that the force is transferred to the corer directly, without dampening the effects of the drill pipe connectors when using uphole percussion. Furthermore, by using the lake water instead of oil as hydraulic fluid to drive the hammer, oil spills are excluded, and hazard-free operations in fragile lake ecosystems are possible.
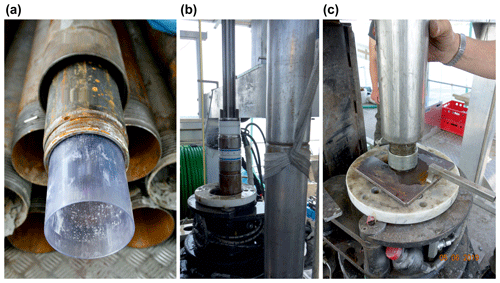
Figure 4Casing, core barrel, and inner liner before setup (a). Coring assembly lowered into the lake with the connection of the core barrel with the DTH hammer atop the rotary unit (b). Heavyweight rods above the hammer unit hold the rotary unit in place through a makeup plate (c).
The 2 m long coring barrel is tipped by a stainless-steel piston at the lower end (Fig. 4a) and harbors a 90 mm diameter and 2 m long plastic core liner to ensure high-quality coring success. The DTH hammer (Fig. 4b) and heavy-weight rods (2 m, 300 kg) on top ensure that the required weight for percussion momentum is sufficient and directed downwards (Fig. 4c). A high-pressure water pump controls the latter, with flow rates of maximum 120 L min−1 and up to 200 bar operating pressure through a reeled hydraulic hose. This hydraulic hose transfers water and hydraulic energy down the hole and is led in parallel to the Kevlar ropes holding the coring unit and allowing additional axial pull forces.
Once the piston coring unit, DTH hammer, and heavyweights are assembled, they are lowered through the moon pool down into the upper reentry cone (1 × 1 m) on top of the casing pipe that sits on the ground plate. This rigging is controlled online, using two underwater cameras mounted to the ground plate. Coring starts when the piston at the end of the core barrel hits the sediment surface. Within the uppermost few meters, the power of its own weight presses the core barrel into the sediment, while further down the hammer produces impulses up to 70 Hz. This drives the corer and spills pumped water out of the core cutter crown to reduce friction between the sediment and core barrel. After 1.85 m, the piston reaches the top of the core barrel where the plastic liner sits. At this position, the piston is locked, and the hydraulic pressure in the system activates the core catcher and closes the liner at the end of the core barrel. The sediment-infilled coring unit is then winched up to the platform, the core is retrieved and the unit is cleaned for the next run (Fig. 5). After each core trip, the outer casing pipe sinks down deeper, either due to its own weight or by being pushed or hammered to the depth of the previous core run once compacted sediment is reached. If borehole instabilities occur and sediment narrows the borehole to cause stuck casing pipes, water jetting through the holes in the lowered piston can be activated to flush the casing pipe of blocking sediment. In addition, a closed piston system was fabricated and tested at Mondsee to be used once the borehole walls remain steady, and stabilizing casing is not needed anymore.
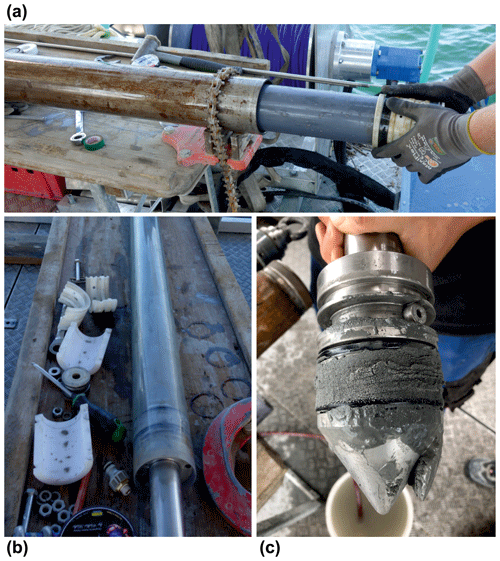
Figure 5Opening of the coring barrel showing a 1.85 m long, sediment-filled plastic liner (gray) and core catcher (white) (a). The piston in the core-filled upper end of the liner (b). The piston after core retrieval, with sandy sediment from Lake Constance (c).
An operating staff for coring and initial core handling of at least four persons are required to run Hipercorig in coring mode. The assembly or dismantling onshore requires 5 d of work, also for a crew of four to five.
3.1 Mondsee
Mondsee is a glaciogenic perialpine lake. Its uppermost sedimentary infill has previously been studied for paleoclimate, paleolimnology, and paleoecology since the Bølling–Allerød interstadial ∼ 14 ka, as covered by several, up to 14 m long, sediment cores retrieved from the deepest part of the lake at ∼ 60 m water depth (Schultze and Niederreiter, 1990; Lauterbach et al., 2011; Swierczynski et al., 2013; Namiotko et al., 2015; Andersen et al., 2017; Daxer et al., 2018). Reflection seismic data, however, imaged a well-stratified, undisturbed postglacial sedimentary sequence of at least 60 m thickness (Fig. 6; Daxer et al., 2018) deposited within a rather short time period of ∼ 4–5000 years after the Traun glacier had retreated from the Mondsee area at about 19 ka (Ivy-Ochs et al., 2008; Reitner, 2011). This scientific setting thus provided an ideal local test site; moreover, the nearby location of the manufacturer's workshops (Uwitec GmbH) in Mondsee village, Austria, eased logistics.
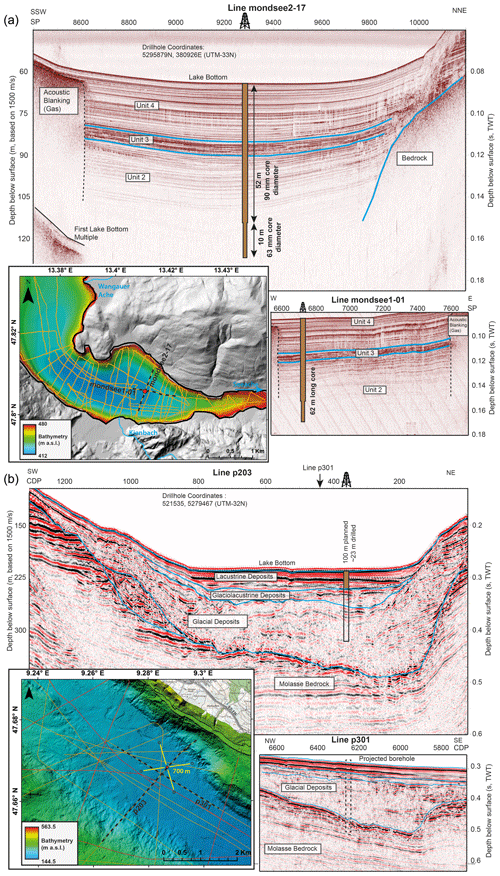
Figure 6Lakes Mondsee and Constance site survey data and coring sites. (a) Bathymetric overview of Mondsee (Austria; data courtesy of Immo Trinks), with the digital elevation model superimposed. Largest (dis-)tributaries are outlined. Orange lines indicate the 3.5 kHz high-resolution single channel seismic tracks. The extent of the seismic profiles is shown as dotted black lines. Seismic interpretation follows Daxer et al. (2018), where all details can be found. (b) Bathymetric overview of Lake Constance, east of Hagnau, with multichannel reflection seismic lines superimposed, recorded in 2016 (red) and in 2017 (orange), using two chamber Mini GI and GI 210 air guns, respectively (unpublished data). Drilling location is given as a red dot, anchor locations are in green, and the seismic stratigraphic horizons are blue lines in Lines p201 and p301, respectively (including borehole locations). The extent of the seismic profiles is shown as a dotted black lines.
Design, building, assembly, and acquisition of parts of the Hipercorig system were conducted in 2016 and 2017, while component assembly was performed during fall of 2017. A first test of the complete system was conducted from 16 April to 1 October 2018 near the previous long coring site, by Daxer et al. (2018) at 60 m water depth (47∘40′565′′ N, 9∘17′12682′′ E), where reflection seismic data show clear coring targets up to 60 m below the lake floor (b.l.f.; Fig. 6a). The assembly of all parts, including the barge with all equipment, was performed within 6 d. On 27 April, the test drive for the nautical certificate (known as the “Schifffahrtstechnisches Gutachten”) was achieved successfully.
After anchoring and site set up, a first adaptation of the coring process and platform layout was necessary. While it was originally planned to use the casing pipes with a reentry cone for the coring device on top, it turned out that it was possible to connect the casing pipes directly from the lake bottom to the platform without a reentry funnel in water depths below 60 m. With this adaptation, the ground plate was assembled, lowered to the lake floor, and connected with the casing and core barrel unit by Kevlar ropes to the platform. As the cameras, tilt sensors, and remote control of the casing brackets worked perfectly, sediment coring began on 9 May 2018. Because of its own weight, the casing pipe sank to the depth reached after each core retrieval up to 10 m b.l.f. Consequently, hole reaming after every 2 m core section was not necessary. At a depth of 26 m b.l.f., operations were halted to solve constructional issues including wear-free seals and a new twist-proof construction of the entire DTH. In addition, dismantling of the entire 86 m (26 m sediment depth plus 60 m water depth) casing pipe was needed to control the crown seal.
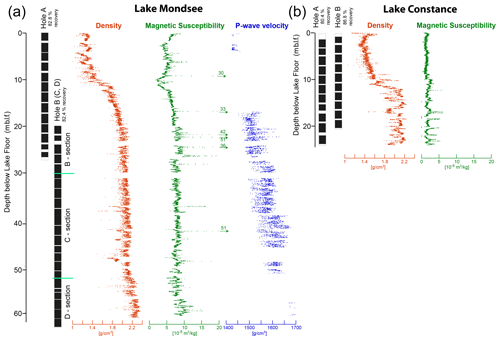
Figure 7Core recovery (a) and physical property data by multisensor core logger (MSCL) (b) data for the composite cores from lakes Mondsee (above) and Constance (below).
During the operational pause, the platform moved slightly away from the ground plate due to wave action, so it was not possible to reenter into the ground plate. In order to avoid such shifts during future operations, a best practice was developed to correct the position with the anchor winches, using the inclination sensors. From late June 2018 on, coring commenced from a minimally offset position (Hole B) from 20 to 30 m depth after displacing the upper 20 m in noncoring mode. Coring in Hole B was continued from 28 m b.l.f., using a manual 70 kg in-hole hammer. However, it took 2 h and 4000 strokes to reach 30 m b.l.f., and 4 t traction was needed to pull out the coring assembly while the casing could neither be progressed nor retrieved. Consequently, it was necessary to invent a new method to continue coring without a casing pipe. For this purpose, the end piece of the coring chamber was modified so that the piston was hydraulically locked, using a valve, until the core filled the chamber and locking could be released. This modification allowed us to reach 52 m b.l.f. coring depth in Hole B (termed section C in Fig. 7). Further tests with different diameter coring (63 and 90 mm) below 52 m b.l.f. coring depth (termed section D) in Hole B were conducted in September 2018 to finally reach a 63 m b.l.f. total coring depth.
The cores retrieved were sealed with end caps in 2 m long clear plastic liners and marked onboard before being stored at an ambient temperature at Uwitec Mondsee and then transported to the Austrian Core Facility (ACF) at the University of Innsbruck for curation and further analysis. Overall core recovery, as calculated from the effective length of sediment-filled core liners compared to the actual penetration advance of the bit (Fig. 7), ranged between 82 % and 78 % for Hole A and B, respectively. Apart from the coring intervals with technical challenges as described above, where core recovery dropped to 50 %, most coring advances achieved nearly 90 % of core recovery. This slightly reduced recovery is due to a systematic loss of core of about 15 cm in the core catcher at the bottom of each run.
At the ACF, cores were further cut into 1 m sections and measured for physical properties (bulk density, P-wave velocity, and magnetic susceptibility) at 0.5 cm resolution, using a Geotek Ltd. multisensor core logger (MSCL). Selected core sections representing different lithofacies, as interpreted from MSCL data (see Sect. 4.1), were split, line-scan photographed and described, and sediment from the lowermost core section, between 62.0 and 62.8 m b.l.f., was sieved to sample terrestrial leaf fragments and cuticles of macroremains for accelerator mass spectrometry (AMS) 14C analysis at the Ion Beam Physics Laboratory of ETH Zürich (ages presented here were calibrated using the IntCal20 calibration curve; Reimer et al., 2020). Furthermore, and for assessing core quality and lithology in 3D, selected core segments were also scanned for X-ray computed tomography (CT), using a Siemens SOMATOM Definition AS at the Medical University of Innsbruck, with a voxel size of 0.2 × 0.2 × 0.3 mm3. Unopened core sections remain stored at 4 ∘C at the ACF and are awaiting further scientific analyses.
3.2 Lake Constance
The lacustrine infill of Lake Constance carries distinctive paleoenvironmental signals (Wessels, 1998; Schwalb et al., 2013) which, so far, could only be recovered from the upper ∼ 11 m thick sediments, although more than ∼ 100 m infill has been interpreted from seismic data (Müller and Gees, 1968). New surveys indicate up to 250 m of Quaternary sediments (Fabbri et al., 2018). The well-defined and horizontally bedded lacustrine sediments of the upper tenth of the meters drilled were thought to be most likely Holocene deposits that overlay the deeper undisturbed Late Glacial sediments. In order to shed new light on the lake as a paleoenvironmental archive and to test Hipercorig in deep lake waters, a second Hipercorig test drilling campaign took place from May to July 2019 at Lake Constance. Site selection was based on a new reflection seismic profiling campaign from 2017, with a focus on the up to 250 m deep central part of the lake (Fabbri et al., 2018, Fig. 6b). A site located ∼ 2 km southwest of Hagnau, Germany (Fig. 6b), was selected based on seismically undisturbed and at least 100 m deep strata in 204 m water depth that is away from the ferry routes and underwater cables but close to a harbor.
The coring campaign began on 13 May 2019 with the setup of Hipercorig in Langenargen harbor, 20 km east of Hagnau. The platform was taxied on 22 May, after a weather delay, to the preselected position for the dropping of the four anchors (Fig. 6). The platform was winched precisely on-site, and the ground plate was lowered to the lake floor in 204 m water depth. The casing was connected on the next day but sank without control due to a failure of the casing winch; it hit the ground plate and damaged the reentry cone. Using an ROV deployment with research vessel (R/V) Kormoran of the Institut für Seenforschung on 28 May 2019, the damage was inspected via video and the ground plate recovered. On 5 June 2019, the ground plate was replaced with a repaired reentry cone before new casings were assembled and set so that coring Hole A could begin. The first five core runs to 10 m depth were piloted smoothly through clay-rich sediments. Below a depth of ∼ 10 m b.l.f., sandy intercalations prevailed, requiring disassembling of the coring system for cleaning after each coring run. Additionally, sand intrusions in the lowermost part of the hole choked the casing pipe. After each core recovery, a cleaning run was necessary to reach the depth of the previous core. On 13 June, a depth of 24 m b.l.f. was reached, with a total recovery of 80 % (Fig. 7), when the coring unit became stuck within the casing pipe due to sand accumulations in the annulus. In order to avoid future wedged coring devices in the casing, a reverse-circulation mode for the water outflowing from the hammer was built during an operational break. It flushes sediment out of the annulus between the casing and coring assembly. During the break, a thunderstorm with > 100 km h−1 gusts slackened one of the anchors, displaced the platform 12 m away from the ground plate, and bent some casing pipes. The hole was not accessible anymore, so the casing pipe and the ground plate had to be dismantled.
The second hole, Hole B, ∼ 10 m away from the original location, was started on 3 July 2019 at 0.5 m b.l.f. depth to create core overlap with the first hole. Coring to 10.5 m b.l.f. proceeded without any problems, until sediments became sandy again and cleaning the coring device and the well from the mobilized sand became necessary, as in Hole A. Coring continued with slightly slower progress, and a total 87 % core recovery to a depth of 20.5 m b.l.f. was reached. Then, on 8 July, a shackle failure in the rig caused a loss in the coring assembly. On 9 July, the situation underwater was inspected, again using the R/V Kormoran ROV. On 10 July, the ground plate unit and casing pipe were pulled onboard and dismantled and Hole B was abandoned. Finally, three 2 m long surface cores were taken to have a undisturbed sediment–water interface and, 1 d later, all the remaining parts and the anchors were retrieved, and the barge was driven back to Langenargen harbor. Within 5 d, the entire system was dismantled and packed into the four containers for shipping. A total of 21 core runs produced 42 m of core and demonstrated Hipercorig's capability to access deep, coarse, and severely compacted lake sediments.
The cores retrieved were sealed with end caps in the 2 m long clear plastic liners, marked, and curated onboard. After being packed into thermoboxes and cooled with ice, they were brought directly by boat to the microbiology labs of the University of Constance to minimize degradation or loss of volatiles. In the labs, the liners were cut into 1 m long sections. First, small samples were taken for methane and DNA measurements before the liners were sealed again and stored at 4 ∘C. After the end of the field campaign, all cores were transported to the Institute of Geological Sciences at the University of Bern. MSCL core logging, core opening, initial core description, and the sampling party were held in Bern in October 2019. From the marking on the barge, all data were entered into the new drilling information software called mDIS, of the International Continental Scientific Drilling Program (ICDP; Behrends et al., 2020), following the guidelines of international lake-drilling projects in ICDP. This was the first application of mDIS in a field campaign, and it serves as blueprint for future ICDP projects.
4.1 Mondsee
The upper 10 m of the cored lacustrine stratigraphic succession of Mondsee is composed of faintly laminated sediment (the dominant lithology is authigenic micritic carbonate and diatoms with little to no detrital grains), with abundant organic material (revealing rather low-density values < 1.4 g cm−3) and intercalated layers of high detrital input (characterized by elevated magnetic susceptibility (MS) values). Distinctly lower MS values, around 10 m b.l.f., and the rather sharp downcore increase in bulk density, from 1.4 to 1.9 g cm−3 between 10 and 15 m b.l.f. (Fig. 7), match well with the previously reported physical properties across the Late Glacial to Holocene transition from denser mixed detrital to lower density authigenic carbonate with intercalated calcitic detrital layers lithofacies (Daxer et al., 2018; Lauterbach et al., 2011). X-ray computed tomography (XCT) data of cores from these upper 15 m reveal abundant gas cracks (Fig. 8) and core disturbances (the latter due to core handling and noncooled storage of the gas- and organic-rich postglacial sediment cores), which also explains the lack of reliable P-wave velocity data in the upper coring interval (Fig. 7).
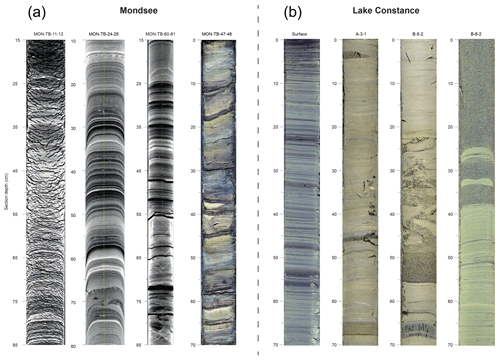
Figure 8(a) Examples from the X-ray computed tomography (XCT) scan (the first three sections from the left; labeled numbers on top of sections indicate subsurface depth range of 1 m core segments) and core photos from Mondsee (histogram-equilibrated images at depth intervals of 47.0–47.7 m b.l.f.) and Lake Constance (four segments to the right). Note the frequent gas expansion cracks in the XCT segment of Mondsee (11.15–11.85 m b.l.f.) and dense (high XCT value in white) conjugated faults at Mondsee (60.80–60.85 m b.l.f), which are further discussed in the text. (b) Four examples of line-scan images from core sections of Lake Constance's composite sections. Depth in centimeters indicates section depths. From left to right: surface core (0–70 cm in meters composite depth – mcd); finely layered brown-gray laminated muds with upcore increasingly darker colors (eutrophication signal); core A-3-1 (5.75–6.45 mcd) – carbonate-rich tan and laminated marls; core B-5-2 (9.75–10.45 mcd) – stiff detrital mud with intercalated sand layers. The middle part of this section shows some core disturbance. Core B-8-2 (15.90–16.60 mcd) – massive sand layers with intercalated thin, graded gray and yellowish silt layers, which indicate an annually laminated loess and/or meltwater record deposited during the Oldest Dryas (Wessels, 1998).
Below 15–20 m b.l.f., XCT data reveal excellent core quality with only minimal deformation drag along the liner wall and no evidence for any coring-induced flow structures in sand layers. The cored succession is composed of laminated detrital carbonates with centimeter-scale fine-grained calcitic turbidites with siliciclastic components, intercalated yellowish detrital layers (poorly sorted medium-sized silt), occasional dropstones, and soft sediment deformation structures (Fig. 8). Density values remain rather constant at ∼ 2 g cm−3, while P-wave velocities increase constantly with depth, from about 1500 to 1650 m s−1. Distinct layers, showing peaks 2 to 5 times higher than the general background values of m3 kg−1-corrected, mass-specific MS, occasionally occur throughout but are more abundant between 20 and 26 m b.l.f. This coring interval correlates to seismic unit 3 by Daxer et al. (2018), interpreted as a phase of increased erosion in the hinterland that is possibly linked to the Gschnitz stadial (Heinrich event 1).
Below 52 m b.l.f., MSCL data are reported with caution because the smaller diameter and partially opened and dried-out core conditions are likely to have influenced the higher density values of up to 2.3 cm3 and P-wave values up to 1700 m s−1 (Fig. 7). The sediment, however, also differs in this lowermost cored succession and is composed of mixed laminated detrital carbonates and up to 1 dm thick fine-grained turbidites. There are distinct, localized, high-density conjugated deformation features (Fig. 8), which cannot be explained by coring artifacts. Instead, they show that the sediment was compressed, possibly by glaciotectonic processes, which would explain the higher density and P-wave values as measured by MSCL.
The radiocarbon age from small leaf fragments and cuticles of macroremains obtained from sieving the sediment of the lowermost 50 cm of the cored interval (sample ETH-105425; 62.3–62.8 m b.l.f.; C14 age – 15 142 ± 41 years BP) reveals a calibrated (95.5 % probability) age of 18 262–18 650 cal BP. This age is well within the range of the dated deglaciation of the region (19 ± 1 ka; Ivy-Ochs et al., 2008; Reitner, 2011) and supports the lithology-based core interpretation that Hipercorig reached glacial sediments and, thus, recovered a complete lacustrine sedimentary succession since the deglaciation of the Mondsee region. The new Mondsee record, unraveled by Hipercorig, represents one of only few that have actually sampled and dated an undisturbed sedimentary sequence since deglaciation in the Alpine foreland (Zübo core; Lister et al., 1988). The record comprises a ∼ 50 m thick, high-resolution glaciolacustrine sedimentary succession that was deposited within the first ∼ 3800 years after deglaciation (between ∼ 18 450 and ∼ 14 670 cal BP; average sedimentation rate ∼ 1.32 cm yr−1)
4.2 Lake Constance
The average core recovery of 80 % and 87 % in Holes A and B, respectively, allows us to construct a gapless composite record from the lake bottom downcore to 14.5 m composite depth (mcd). Below, down to 24.01 m final composite depth, four intervals ranging in thickness between 13 and 40 cm were not recovered, representing minor gaps. This longest ever recovered sedimentary succession from Lake Constance allows a novel look into the paleoenvironmental evolution of the lake well back into the Last Glacial Period. Core quality is high, with minor disturbances usually in the uppermost 20–30 cm of each 2 m run, in particular in the softer upper part of the section. The coring system was able to fully recover lithologies that are usually challenging for any coring technique. These include intercalations of centimeter-scale coarse sand layers with laminated silty intervals or meter-thick sand units (Fig. 8) that are recovered without evident disturbances.
A previously studied core collected at a site nearby reached a depth of 8.7 m (BO97/14; Wessels 1998; Hanisch et al., 2009; Schwalb et al., 2013). Comparing the MSCL-density curve of the new core with the porosity curve of the former core suggests that the sedimentation rate is a bit higher at the new site. The bottom of the 8.7 m long former core, with a basal age of ∼ 17 000 cal BP, may correlate to the new core at ∼ 12 mcd (Fig. 7; Schwalb et al., 2013). The lithologies from the surface to that depth can be correlated to various lithologic units described in previous studies, ranging from brown-gray laminated muds in the uppermost meters (surface core; Fig. 8) to underlying carbonate-rich tan and laminated marls (Core A-3-1; Fig. 8), both representing the Holocene section (Wessels, 1998). Densities in this upper part of the core range between 1.3 and 1.5 g cm−3, with a few coarse-grained and graded layers reaching up to ∼ 1.8 g cm−3 (Fig. 7). Noteworthy is the recent upcore increase in organic matter towards the surface, with dark blackish colors reflecting anthropogenic eutrophication (surface core; Fig. 8; Wessels, 1998; Wessels et al., 1999; Blattmann et al., 2020).
These Holocene units are underlain by massive (density up to ∼ 2.1 g cm−3) detrital mud with occasional sand layers (Core B-5-2; Fig. 8) deposited during the Younger Dryas and by finely laminated yellow-brown to gray clayey silt, dating further back into the Late Glacial, containing alternating layers of yellow loess and gray meltwater deposits (Niessen et al., 1992; Wessels, 1998). From there, downcore (i.e., from ∼ 12–24 mcd), the recovered lithologies represent a previously unstudied succession that is currently not dated. Sediments consist of layers of structureless coarse sand that may reach bed thicknesses of over 1 m, with density values partly over 2.2 g cm−3 (Fig. 7). These sands are intercalated by fine-grained and laminated silty sections, up to few decimeters thick, consisting of numerous graded millimeter- to centimeter-scale layers (Core B-8-2; Fig. 8). These lithologies represent typical depositional processes in periglacial or proglacial lake–near delta environments that occasionally provide high-energy underflows delivering sand, even to water depths of over 200 m. The laminated finer grained graded layers, in contrast, likely reflect deposition away from active channels and presumably have lower sedimentation rates. The site most probably represents an initial stage of the evolving lake, as described elsewhere (Wessels, 1998; Schwalb et al., 2013), but with a high proportion of river-borne sand, reflecting the interplay of the lake with its catchment during the Last Glacial Period.
Motivated by the lack of cost-effective, deep, continuous, and high-quality coring capabilities in unconsolidated sediments, our primary technological goal of this study was to develop and test a new system that meets these requirements. Although various coring devices have been deployed (Leroy and Colman, 2001) in the past, recent experiences in the framework of ICDP have shown (e.g., Russell et al., 2016) that, for lake sediment thicknesses in the 100 m range, no viable alternative to commercial wire line drilling operations exists so far. Therefore, our goal was to provide a system with a number of key objectives discussed below.
The primary ambition to overcome the usual 20 to 30 m coring depth limit (although exceptional soft sediments in special cases allow coring deeper; e.g., Mingram et al., 2007) was very well achieved, and previous attempts to core deeper in lakes Mondsee and Constance were convincingly outnumbered. In addition, the penetration of hard layers was successfully performed in the stiff glacial sediments of Mondsee and the massive sand intercalations of Lake Constance. This is highly promising for future drilling campaigns in, for example, turbiditic or volcanic ash layers as well. However, these achievements need additional technical modifications, such as a sand cleaning tool and reverse circulation water flow for jetting the annulus free from sand, that added complexity to Hipercorig's operations. Observations using an ROV showed that hydraulic hammering and pumping of large volumes of water had only negligible effects on the lake floor and did not lead to large plumes of turbid water which might affect sensitive organisms.
The target depth of 100 m coring penetration has not been achieved. However, the 63 m maximum depth reached provided an excellent prospect for matching the initial goal in a variety of sedimentary basins. Nevertheless, future Hipercorig projects still need to tackle this challenge. On the other hand, operations at 204 m water depths were performed despite major challenges. The strong water currents in Lake Constance caused deviations in the coring system from the ground plate that call for control of reentry via either ROV or an additional upward-looking underwater camera at the reentry cone. A concept for using the reentry cone and ground plate system for geophysical wire line logging has been developed but could, so far, not be tested and awaits deployment in future missions. The mooring works well for calm conditions but enhanced anchoring will be needed in storms.
Core quality and continuity are as convincing as with other well-proven modern coring systems, while the perseverance to hitherto inaccessible depths is convincing. However, it must be noted that there is a limit of core recovery due to the inevitable loss of core catcher material at the lower end of the corer. Therefore, approximately 90 % core recovery is a realistic upper limit. The way to achieve full coverage is by double coring with a second hole at the same drill site. This remains an immense cost and time factor, as it is for all other systems at this point. However, the effective costs of operating Hipercorig at Lake Constance were a factor of 10 times lower than comparable wire line coring operations in similar environments and consequently provided an outstanding opportunity for the cost-effective coring of unconsolidated deposits. The limitations of Hipercorig's operational range, as experienced with the experiments in the two perialpine lakes, are (i) strongly compacted sediment layers over several tens of meters and (ii) strong wave actions over 1 m and winds over 8 Bt that require a halt in operations with temporary evacuation.
Hipercorig is a novel, high-quality soft sediment coring system. Its performance has been demonstrated on perialpine lakes in over 200 m water depths and more than 60 m coring depth of lacustrine and glacial sediments. It offers promising prospects for similar environments, including targets in shallow marine environments. The new Mondsee core, obtained by Hipercorig, represents the first core that sampled, without extensive commercial drilling efforts, a complete and undisturbed sedimentary sequence since the deglaciation of the Alpine foreland. It also allowed recovery of material for 14C dating and framing the age of deglaciation of the Mondsee region to 18.456 ± 194 cal BP. The new Lake Constance core comprises Holocene laminated muds and marls underlain by massive detrital muds and intercalated sand layers of the Younger Dryas and alternating finely laminated silt, loess, and meltwater deposits from the Oldest Dryas. This is underlain by previously unsampled thick coarse sand beds and intercalated fine silts, showing a dynamic distal delta development adjacent to an Alpine glacier.
Data sets used in this article are available upon request. The data sets will be published independently in an open access data publication as soon as they are complemented by further laboratory data.
UH, AS, and VW designed the study. RN and VW designed Hipercorig. RN performed coring on Mondsee. RN, with UR, AS, and MW, led the expedition on Lake Constance. MS directed the seismic campaign on Mondsee and investigated the Mondsee cores. FSA, SCF, and SeS conducted and interpreted the seismic profiling and investigated the cores from Lake Constance. UH, UR, MS, and FSA were the primary authors of the paper, with input from all other coauthors.
The first author is an editor of Scientific Drilling; all other authors declare that they have no conflict of interest.
We are grateful for the financial support from the Deutsche Forschungsgemeinschaft and the German Scientific Earth Probing (GESEP) consortium for the building and testing of Hipercorig. We deeply appreciate the dedicated work of the Uwitec staff who built Hipercorig and conducted operations. Administrative and logistical support for the Lake Constance operations was provided by Landratsamt Bodenseekreis, Schifffahrtsamt, in Friedrichshafen and the Hagnau local authorities. We would also like to thank the many participants from Universität Konstanz, Anja Grießer for the MSCL measurements of Mondsee cores at the Austrian Core Facility, University of Innsbruck, and Christoph Daxer for the sampling material for radiocarbon dating and discussion of Mondsee data, results, and interpretations.
This research has been supported by the Deutsche Forschungsgemeinschaft (grant nos. SCHW 671/19-1, BR 3573/3-1, and 290492639).
This paper was edited by Jan Behrmann and reviewed by Laurent Augustin and Bernd Zolitschka.
Andersen. N., Lauterbach, S., Erlenkeuser, H., Danielopol, D. L., Namiotko, T., Hüls, M., Belmecheri, S., Dulski, P. Nantke, C., Meyer, H., Chapligin, B., von Grafenstein, U., and Brauer, A.: Evidence for higher-than-average air temperatures after the 8.2 ka event provided by a Central European δ18O record, Quaternary Sci. Rev., 172, 96–108, https://doi.org/10.1016/j.quascirev.2017.08.001, 2017.
Behrends, K., Heeschen, K., Kunkel, C., and Conze, R.: The mobile Drilling Information System (mDIS) for core repositories, EGU General Assembly 2020, Online, 4–8 May 2020, EGU2020-13663, https://doi.org/10.5194/egusphere-egu2020-13663, 2020.
Blattmann, T., Lesniak, B., García-Rubio, I., Charilaou, M., Wessels, M., Eglinton, T. I., and Gehring, A. U.: Ferromagnetic resonance of magnetite biominerals traces redox changes, Earth Planet. Sc. Lett., 545, 11640, https://doi.org/10.1016/j.epsl.2020.116400, 2020.
Brigham-Grette J., Melles M., Minyuk P., Andreev A., Tarasov P., DeConto R., Koenig S., Nowaczyk N., Wennrich V., Rosén P., Haltia E., Cook T., Gebhardt C., Meyer-Jacob C., Snyder J., and Herzschuh U.: Pliocene warmth, polar amplification, and stepped Pleistocene cooling recorded in NE Arctic Russia, Science, 340, 1421–1427, https://doi.org/10.1126/science.1233137, 2013.
Daxer, C., Moernaut, J., Haas, J., Strasser, M., and Taylor, T.: Late Glacial and Holocene sedimentary infill of Lake Mondsee (Eastern Alps, Austria) and historical rockfall activity revealed by reflection seismics and sediment core analysis, Austrian J. Earth Sc., 111, 111–134, https://doi.org/10.17738/ajes.2018.0008, 2018.
Fabbri S. C., Allenbach, R., Herwegh, M., Krastel, S., Lebas, E., Lindhorst, K., Madritsch, H., Wessels, M., Wielandt-Schuster, U., and Anselmetti F. S.: Bedrock structure, postglacial infill and neotectonic fault structures in Lake Constance, 16th Swiss Geoscience Meeting, 30 November–1 December 2018, Bern, Switzerland, 2018.
Gallmetzer I., Haselmair A., Stachowitsch M., and Zuschin M.: An innovative piston corer for large-volume sediment samples, Limnol. Oceanogr.-Meth., 14, 698–717, https://doi.org/10.1002/lom3.10124, 2016.
Hanisch, S., Wessels, M., Niessen, F., and Schwalb, A.: Late Quaternary lake response to climate change and anthropogenic impact: biomarker evidence from Lake Constance sediments, J. Paleolim., 41, 393–406, https://doi.org/10.1007/s10933-008-9232-4, 2009.
Ivy-Ochs, S., Kerschner, H., Reuther, A., Preusser, F., Heine, K., Maisch, M., Kubik, P. W., and Schlüchter, C.: Chronology of the last glacial cycle in the European Alps, J. Quaternary Sci., 23, 559–573, https://doi.org/10.1002/jqs.1202, 2008.
Johnson, T. C., Werne, J. P., Brown, E. T., Abbott, A., Berke, M., Steinman, B. A., Halbur, J., Contreras, S., Grosshuesch, S., Deino, A., Scholz, C. A., Lyons, R. P., Schouten, S., and Sinninghe Damste, J. S.: A progressively wetter climate in southern East Africa over the past 1.3 million years, Nature, 537, 220–224, https://doi.org/10.1038/nature19065, 2016.
Lauterbach, S., Brauer, A., Andersen, N., Danielopol, D. L., Dulski, P., Hüls, M., Milecka, K., Namiotko, T., Obremska, M., and Von Grafenstein, U.: Environmental responses to Lateglacial climatic fluctuations recorded in the sediments of pre-Alpine Lake Mondsee (northeastern Alps), J. Quaternary Sci., 26, 253–267, https://doi.org/10.1002/jqs.1448, 2011.
Leroy, S. A. G. and Colman, S. M.: Coring and drilling equipment and procedures for recovery of long lacustrine sequences, in: Tracking Environmental Change Using Lake Sediments, Volume 1: Basin Analysis, Coring, and Chronological Techniques, edited by: Last, W. M. and Smol, J. P., Kluwer, Dordrecht, the Netherlands, 2001.
Lister, G. S.: A 15,000-year isotopic record from Lake Zurich of deglaciation and climatic change in Switzerland, Quaternary Res., 29, 129–141, https://doi.org/10.1016/0033-5894(88)90056-7, 1988.
Litt, T. and Anselmetti, F.S.: Lake Van Deep Drilling Project PALEOVAN, Quaternary Sci. Rev., 104, 1–7, https://doi.org/10.1016/j.quascirev.2014.09.026, 2014.
Melles, M., Brigham-Grette, J., Minyuk, P., Nowaczyk, N. R., Wennrich, V., DeConto, R. M., Anderson, P. M, Andreev, A. A., Coletti, A., Cook, T. M., Haltia-Hovi, E., Kukkonen, M., Lozhkin, A. V., Rosen, P., Tarasov, P., Vogel, H., and Wagner, B.: 2.8 Million Years of Arctic Climate Change from Lake El'gygytgyn, NE Russia, Science, 337, 315–320, https://doi.org/10.1126/science.1222135, 2012.
Mingram, J., Negendank, J. F., Brauer, A., Berger, D., Hendrich, A., Köhler, M., and Usinger, H.: Long cores from small lakes – recovering up to 100 m-long lake sediment sequences with a high-precision rod-operated piston corer (Usinger-corer), J. Paleolimnol., 37, 517–528, 2007.
Müller, G. and Gees, R. A.: Origin of the Lake Constance Basin, Nature, 217, 836–837, 1968.
Namiotko, T., Danielopol, D. L., von Grafenstein, U., Lauterbach, S., Brauer, A., Andersen, N., Hüls, M., Milecka, K., Baltanás, A., Geiger, W., Belmecheri, S., Desmet, M., Erlenkeuser, H., and Nomade, J.: Palaeoecology of late glacial and Holocene profundal Ostracoda of pre-Alpine lake Mondsee (Austria) – A base for further (palaeo-) biological research, Palaeogeogr. Palaeocl., 419, 23–36, https://doi.org/10.1016/j.palaeo.2014.09.009, 2015.
Niessen, F., Lister, G., and Giovanoli, F.: Dust transport and palaeoclimate during the oldest Dryas in Central Europe – implications from varves (Lake Constance), Clim. Dynam., 8, 71–81, https://doi.org/10.1007/BF00209164, 1992.
Reimer, P., Austin, W., Bard, E., Bayliss, A., Blackwell, P., Bronk Ramsey, C., Butzin, M., Cheng, H., Lawrence Edwards, R., Friedrich, M., Grootes, P. M., Guilderson, T. P., Hajdas, I., Heaton, T. J., Hogg, A. G., Hughen, K. A., Kromer, B., Manning, S. W., Muscheler, R., Palmer, J. G., Pearson, C., van der Plicht, J., Reimer, R., Richards, D. A., Scott, E. M., Southon, J. R., Turney, C. S. M., Wacker, L., Adolphi, F., Büntgen, U., Capano, M., Fahrni, S., Fogtmann-Schulz, A., Friedrich, R., Miyake, F., Olsen, J., Reinig, F., Sakamoto, M., Sookdeo, A., and Talamo, S.: The IntCal20 Northern Hemisphere Radiocarbon Age Calibration Curve (0–55 cal kBP), Radiocarbon, 62, 725–757, https://doi.org/10.1017/RDC.2020.41, 2020.
Reitner, J. M.: Das Inngletschersystem während des Würm-Glazials, in: Arbeitstagung der Geologischen Bundesanstalt, 19–23 September 2011, Achenkirch, Austria, 79–88, https://doi.org/10.13140/RG.2.1.3754.1520, 2011.
Russell, J. M., Bijaksana, S., Vogel, H., Melles, M., Kallmeyer, J., Ariztegui, D., Crowe, S., Fajar, S., Hafidz, A., Haffner, D., Hasberg, A., Ivory, S., Kelly, C., King, J., Kirana, K., Morlock, M., Noren, A., O'Grady, R., Ordonez, L., Stevenson, J., von Rintelen, T., Vuillemin, A., Watkinson, I., Wattrus, N., Wicaksono, S., Wonik, T., Bauer, K., Deino, A., Friese, A., Henny, C., Imran, Marwoto, R., Ngkoimani, L. O., Nomosatryo, S., Safiuddin, L. O., Simister, R., and Tamuntuan, G.: The Towuti Drilling Project: paleoenvironments, biological evolution, and geomicrobiology of a tropical Pacific lake, Sci. Dril., 21, 29–40, https://doi.org/10.5194/sd-21-29-2016, 2016.
Schultze, E. and Niederreiter, R.: Paläolimnologische Untersuchungen an einem Bohrkern aus dem Profundal des Mondsees (Oberösterreich), Linzer Biologische Beiträge, 22, 213–235, 1990.
Schwalb, A., Dean, W.E., Güde, H., Hanisch, S., Sobek, S., and Wessels, M.: Benthic ostracode ∂13C as sensor for early Holocene establishment of modern circulation patterns in Central Europe, Quaternary Sci. Rev., 66, 112–122, https://doi.org/10.1016/j.quascirev.2012.10.032, 2013.
Swierczynski, T., Lauterbach, S., Dulski, P., Delgado, J., Merz, B., and Brauer, A.: Mid- to late Holocene flood frequency changes in the northeastern Alps as recorded in varved sediments of Lake Mondsee (Upper Austria), Quaternary Sci. Rev., 80, 78–90, https://doi.org/10.1016/j.quascirev.2013.08.018, 2013.
Wagner, B., Vogel, H., Francke, A., Friedrich, T., Donders, T., Lacey, J. H., Leng, M. J., Regattieri, E., Sadori, L., Wilke, T., Zanchetta, G. Albrecht, C., Bertini, A., Combourieu-Nebout, N., Cvetkoska, A., Giaccio, B., Grazhdani, A. Hauffe, T., Holtvoeth, J., Joannin, S., Jovanovska, E., Just, J., Kouli, K., Kousis, I., Koutsodendris, A., Krastel, S., Lagos, M., Leicher, N., Levkov, Z., Lindhorst, K., Masi, A., Melles, M., Mercuri, A. M., Nomade, S., Nowaczyk, N., Panagiotopoulos, K., Peyron, O., Reed, J. M., Sagnotti, L., Sinopoli, G., Stelbrink, B., Sulpizio, R., Timmermann, A., Tofilovska, S., Torri, P., Wagner-Cremer, F., Wonik, T., and Zhang, X.: Mediterranean winter rainfall in phase with African monsoons during the past 1.36 million years, Nature, 573, 256–260, https://doi.org/10.1038/s41586-019-1529-0, 2019.
Wessels, M.: Natural environmental changes indicated by Late Glacial and Holocene sediments from Lake Constance, Germany, Palaeogeogr. Palaeocl., 140, 421–432, https://doi.org/10.1016/S0031-0182(98)00026-1, 1998.
Wessels, M., Mohaupt, K., Kümmerlin, R., and Lenhard, A.: Reconstructing Past Eutrophication Trends from Diatoms and Biogenic Silica in the Sediment and the Pelagic Zone of Lake Constance, Germany, J. Paleolimnol., 21, 171–192, https://doi.org/10.1023/A:1008080922586, 1999.
Wessels, M., Anselmetti, F., Artuso, R., Baran, R., Daut, G., Geiger, A., Gessler, S., Hilbe, M., Möst, K., Klauser, B., Niemann, S., Roschlaub, R., Steinbacher, F., Wintersteller, P., and Zahn, E.: Bathymetry of Lake Constance – State of the Art in Surveying a Large Lake, Hydrographische Nachrichten, 100, 6–11, 2015.
- Abstract
- Introduction
- Key components and functions of Hipercorig
- Test sites, operations, and methodology
- Initial core description, measurements, and results
- Discussion
- Conclusions
- Data availability
- Author contributions
- Competing interests
- Acknowledgements
- Financial support
- Review statement
- References
- Abstract
- Introduction
- Key components and functions of Hipercorig
- Test sites, operations, and methodology
- Initial core description, measurements, and results
- Discussion
- Conclusions
- Data availability
- Author contributions
- Competing interests
- Acknowledgements
- Financial support
- Review statement
- References