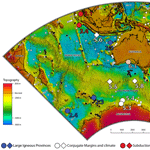
Developing community-based scientific priorities and new drilling proposals in the southern Indian and southwestern Pacific oceans
Neville Exon
Dietmar Müller
Karsten Gohl
Michael Gurnis
Amelia Shevenell
Stuart Henrys
Fumio Inagaki
Dhananjai Pandey
Jessica Whiteside
Tina van de Flierdt
Tim Naish
Verena Heuer
Yuki Morono
Millard Coffin
Marguerite Godard
Laura Wallace
Shuichi Kodaira
Peter Bijl
Julien Collot
Gerald Dickens
Brandon Dugan
Ann G. Dunlea
Ron Hackney
Minoru Ikehara
Martin Jutzeler
Lisa McNeill
Sushant Naik
Taryn Noble
Bradley Opdyke
Ingo Pecher
Lowell Stott
Gabriele Uenzelmann-Neben
Yatheesh Vadakkeykath
Ulrich G. Wortmann
An International Ocean Discovery Program (IODP) workshop was held at Sydney University, Australia, from 13 to 16 June 2017 and was attended by 97 scientists from 12 countries. The aim of the workshop was to investigate future drilling opportunities in the eastern Indian Ocean, southwestern Pacific Ocean, and the Indian and Pacific sectors of the Southern Ocean. The overlying regional sedimentary strata are underexplored relative to their Northern Hemisphere counterparts, and thus the role of the Southern Hemisphere in past global environmental change is poorly constrained. A total of 23 proposal ideas were discussed, with ∼ 12 of these deemed mature enough for active proposal development or awaiting scheduled site survey cruises. Of the remaining 11 proposals, key regions were identified where fundamental hypotheses are testable by drilling, but either site surveys are required or hypotheses need further development. Refinements are anticipated based upon regional IODP drilling in 2017/2018, analysis of recently collected site survey data, and the development of site survey proposals. We hope and expect that this workshop will lead to a new phase of scientific ocean drilling in the Australasian region in the early 2020s.
- Article
(6474 KB) - Full-text XML
- BibTeX
- EndNote
The importance of the Southern Hemisphere in the narratives of global plate tectonics and oceanography is well established, but understudied. This is in large part due to the vastness of the eastern Indian Ocean, southwestern Pacific Ocean, and the Indian and Pacific sectors of the Southern Ocean. This is an ideal region to address many of the 14 science challenges in the 2013–2023 IODP science plan. The Australian and Indian continents have undergone the largest and most rapid paleo-latitudinal shifts of any continents globally since 150 Ma. The region boasts the following: (i) arguably the greatest diversity of subduction zones from fully seismically coupled to uncoupled; (ii) extensive shallow marine seas and submerged continents (e.g., Zealandia) with extraordinary and unstudied stratigraphic records; and (iii) the largest suite of plume-related products and the largest mantle cold spot. Sampling of plateaus, ridges, and their associated sedimentary strata will provide an enormous wealth of information about their origin and address fundamental paleoceanographic and paleoclimate questions. Drilling of the Antarctic margin in the Indian Ocean and South Pacific sectors will increase our understanding of the Antarctic cryosphere and global climate evolution and past land and sea ice extent from the Cretaceous through the Cenozoic. Geomicrobiological questions can be addressed on a number of expeditions, including targeted expeditions to study the deep biosphere in a variety of tectonic settings. Petrological and geochemical studies of oceanic, back-arc and arc crust, as well as uplifted mantle remain a high priority, as do those of geological hazards.
To facilitate and nurture cross-disciplinary proposals, workshop breakout sessions focused on distinct tectonic settings and their associated paleo-environmental evolution. These included (1) large igneous provinces and associated paleoceanography, (2) subduction zones and associated paleoceanography, (3) a separate focus group on the Hikurangi subduction zone, (4) conjugate margin/Zealandia studies and associated paleoceanography, and (5) a biosphere frontiers subgroup meeting not related to the above tectonic settings. The potential proposals discussed in the breakout sessions are listed in Table 1, and locations shown in Fig. 1.
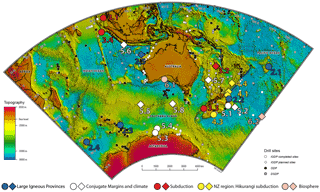
Figure 1Location map of potential proposals discussed in the workshop, with color-coded dots denoting the main theme for each proposal. Small colored circles indicate previous (and planned) drilling by the Deep Sea Drilling Project (DSDP), Ocean Drilling Program (ODP), and IODP. Larger circles and diamonds are projects discussed in the meeting and are colored by theme, and numbered according to sections discussed in text. Large circles indicate proposals that appear mature enough to develop pre-proposals. Large diamonds require site survey proposals to be developed, or are awaiting the results of upcoming drilling in the region (e.g., Hikurangi subduction zone and Lord Howe Rise regions) and may require a focus workshop to further refine hypotheses.
Earth's evolution includes multiple, geologically brief episodes when extraordinary volcanism occurred across some surface regions. Documentation for this comes from large igneous provinces (LIPs), extensive areas covered by thick layers of mostly mafic material that was emplaced on million-year timescales. While LIPs have been widely acknowledged and discussed by the geoscience community for more than two decades, major first-order questions regarding their origin and environmental impact remain. Profound and rapid changes in biota and chemical cycling have also punctuated Earth's history and many of these ”events” have been linked to the formation of LIPs. For example, massive volcanic outpouring may have been coupled to large increases in atmospheric pCO2, which could have raised surface temperatures, amplified the hydrological cycle, and changed ocean circulation. Equally important is the fact that oceanic LIPs typically lie above the carbonate compensation depth, thereby providing the elevated foundation on which many outstanding records of Earth's climate history accumulate.
2.1 Manihiki-Plus: ground zero for understanding large igneous provinces and their environmental impact
Manihiki Plateau, in the southwestern Pacific, is a large (770 000 km2) bathymetric high, which appears to have been emplaced about 125–120 Ma. Five drill sites are proposed, four on Manihiki Plateau and one on the older Magellan Rise. Numerous hypothesis could consequently be addressed: (1) Manihiki Plateau was part of a much larger LIP that has been disjointed since the Cretaceous (other components are believed to include the Ontong Java and Hikurangi plateaus); (2) the environmental impacts of LIP emplacement can be monitored by syn-LIP sedimentation at proximal older locations, especially Magellan Rise; and (3) the sedimentary records on top of Manihiki Plateau and Magellan Rise hold the paleoceanographic history of the Central Pacific from the Late Jurassic to present, as the two locations lie beneath the eastern end of the Pacific warm pool.
2.2 Hikurangi Plateau large igneous province
Drilling on the Hikurangi Plateau will yield insights into the mantle source and LIP emplacement rates, and help to constrain geodynamic models and environmental impacts of LIP emplacement. It will also enable testing of the hypothesis that Ontong Java, Manihiki, and Hikurangi were once part of a single super-LIP, and will allow controls on subduction megathrust slip behavior to be studied. Upcoming drilling on IODP expeditions 372 and 375 will provide critical information to underpin the development of such a proposal, as will multichannel seismic reflection and refraction lines to be acquired in November/December of this year. It was suggested that the proponent group aim to develop a pre-proposal by October 2018, once all of the information is available and hypotheses could be fully formulated.
2.3 Kerguelen Plateau large igneous province emplacement and associated paleoceanography
A multidisciplinary drilling expedition on the Kerguelen Plateau will investigate LIP formation and Southern Ocean paleoceanography over the past 120 Ma. The Kerguelen Plateau incorporates multiple microcontinents, and has as unknown relationship to dipping reflection sequences on the nearby Antarctic margin. Tectonomagmatic questions include why the most voluminous magmatism appears to have post-dated continental breakup (unlike other flood basalts associated temporally with breakup), and how continental fragments were isolated in the plateau. Cretaceous and Cenozoic paleoceanographic records are well preserved in regional carbonates, and the complex topography of the Kerguelen Plateau exerts a strong influence on the pathways of water masses within the Antarctic Circumpolar Current (ACC) and the Antarctic Bottom Water (AABW). In the Cenozoic era, the pathways and intensities of Southern Ocean circulation were developed and significantly modified by emplacement of the Kerguelen Plateau and opening of regional tectonic gateways.
2.4 Plio-Pleistocene paleoceanography of the southwestern Indian sector of the Southern Ocean (PePSI-SO)
A preliminary proposal (918-Pre) to drill in the Conrad Rise and Del Caño Rise regions (Indian Ocean sector) of the Southern Ocean (SO) was submitted to IODP in April 2017. Five high sediment accumulation sites are proposed, with the aim to document Southern Ocean variability and atmosphere, ocean, and cryosphere interactions in the southwestern Indian Ocean sector. The targeted drill sites will fill important gaps in our knowledge covering the middle Miocene cooling (∼ 14 Ma), late Miocene carbon shift (8–6 Ma), the Pliocene climate optimum (5.3–3.3 Ma), the late Pliocene global cooling (3.3–2.6 Ma), the mid-Pleistocene transition (MPT: 1250–700 ka), and the mid-Brunhes transition (∼ 0.43 Ma). These sites will contribute to further understanding dynamic fluctuations of the ACC and associated meridional frontal migrations in relation to global circulation (e.g., Agulhas Leakage, and Atlantic meridional overturning circulation). Also investigated will be changes in inter-ocean surface and deep-water transport, and past variability in southern Indian Ocean sea ice extent, with implications for air–sea gas exchange and the partitioning of CO2 between the atmosphere and the ocean interior.
2.5 Wombat in the greenhouse: sampling rare Southern Hemisphere records of Mesozoic environmental change
It is proposed to drill Mesozoic sedimentary sequences on the northeastern Wombat Plateau, on the northernmost continental margin of Australia. Drilling during Ocean Drilling Program (ODP) Leg 122 in 1988 obtained a thick succession of Late Triassic deltaic and shallow marine sediments unconformably overlain by Late Cretaceous pelagic sediments, including records of Oceanic Anoxic Event (OAE) 2 and the Cretaceous–Paleogene boundary. However, recovery was poor in part and new core from the Wombat Plateau will provide a better understanding of early Mesozoic paleoclimate, paleoceanography, and paleoenvironments in the Southern Hemisphere. These sites should provide key Southern Hemisphere data for Late Triassic climate events, including the hypothesized late Norian–Rhaetian increase in atmospheric pCO2, Carnian pluvial event, two bolide impacts, and the far-field effects of the Central Atlantic magmatic province, a LIP contemporaneous with the end-Triassic mass extinction. Late Cretaceous sediments will provide an important deep-water record of dynamic environmental changes immediately prior to the end-Cretaceous mass extinction event.
3.1 Andaman back-arc basin: understanding crustal accretion in a sedimented spreading region
The Andaman back-arc basin was formed by subduction of the Indian plate under the Burmese plate. Linear magnetic anomalies indicate that seafloor spreading in the Andaman basin commenced at ∼ 4 Ma, but anomalies are lacking in some sectors. Drilling in the rift valley of the Andaman back-arc basin will provide important insights on crustal accretion and why pronounced linear magnetic anomalies are absent in this sedimented spreading. Drilling at the Alcock and Sewell seamounts and the inferred location of the oldest oceanic crust will help address several salient regional problems, such as the nature of the crust underlying the Alcock and Sewell seamounts, and the timing of formation and evolution of the Andaman back-arc basin.
3.2 Amphibious drilling of the New Caledonia peridotitic ophiolite, northern Zealandia
The geodynamic evolution of the southwestern Pacific, from Gondwana break-up during the Cretaceous to subduction-dominated tectonism in the Cenozoic, resulted in the obduction of a string of peridotite ophiolites/massifs from the Anita ophiolite in Southern New Zealand to the Papuan Ultramafic Belt ophiolite. The New Caledonian ophiolite is one of the largest obducted peridotitic masses in the world. An amphibious drilling proposal (ADP) will provide a more complete understanding of an obducted deep geological system from a terrestrial setting to its marine extension, which is as close as possible to its unobducted mantle lithosphere counterpart. Drilling onshore and offshore along the New Caledonia ophiolite will allow emplacement mechanisms of mantle-dominated allochthons to be assessed, as well as constraining high- and low-temperature alteration processes. Other objectives could relate to studying archaeal and eubacterial communities that are known to develop in these alkaline systems, while the formation of the world's second largest rimmed carbonate reefs during the Miocene to Quaternary will be investigated. Developing an ADP will require engagement of the scientific communities associated with IODP and the International Continental Scientific Drilling Program (ICDP).
3.3 Testing geodynamic models for subduction initiation, mega-thrust development, and deep biosphere development in the Puysegur Trench, south of New Zealand
The Puysegur incipient subduction zone south of New Zealand is an ideal location to constrain key geodynamic unknowns. Precise plate tectonic constraints along with a high level of seismicity reveal the transition of strike-slip motion along the Macquarie Ridge in the south to a clear Benioff zone and active subduction beneath southwestern South Island of New Zealand, in the north. It is likely that the Puysegur subduction zone is currently transitioning from a forced to a self-sustaining state. IODP drilling around Puysegur will allow testing and refinement of three topics fundamental to the IODP science plan 2013–2023: (1) the forces associated with subduction initiation, (2) the origin of subseafloor communities in the deep biosphere, and (3) the development of fault properties in a mega-thrust environment. Site survey data at Puysegur will be acquired with the R/V Marcus Langseth during February to March 2018 through the South Island Subduction Initiation Experiment (SISIE). Numerous associated paleoceanographic objectives will be addressed by drilling in this region, including a record of terrestrial runoff from New Zealand as the convergent plate boundary evolved, and records of sub-Antarctic sea surface conditions and paleoproductivity.
3.4 Stress state in the upper oceanic crust in a region of great intraplate earthquakes off Sumatra
The world's largest known intraplate earthquakes have occurred in the subducting Indian Plate offshore Sumatra, and have raised many questions about the genesis of such events. IODP Expedition 362 had two prospective sites approved for drilling (SUMA-22A and SUMA-23A) located 10–20 km to the north and south of the epicenter of one of these major intraplate earthquakes. Although approved for drilling, the sites were not drilled during Expedition 362 due to time constraints. However, these sites provide a unique opportunity to investigate the stress state in the region of these great intraplate earthquakes, and also to advance understanding of the sedimentary sequence entering the Sumatra subduction zone farther north, thereby building on the goals of Expedition 362. Depending on the scope, either an APL or a full proposal is planned to follow up on Expedition 362 objectives, and to investigate the state of stress state in upper oceanic crust near these highly seismogenic fracture zones.
4.1 Hikurangi subduction inputs
The Hikurangi margin of New Zealand is arguably one of the best locales on the planet to resolve controls on subduction megathrust slip behavior due to the strong along-strike variations in subduction interface slip behavior. The nature of the material entering the subduction zone on the subducting Pacific Plate likely exerts a strong control on these along-strike variations in slip behavior. This proposal will acquire cores and logs sampling the incoming sedimentary section and underlying Hikurangi Plateau at several sites along the Hikurangi Plateau (from north to south). These sites will illuminate along-strike variations in the sedimentary section and underlying Hikurangi Plateau, and how these variations in lithology and fluid content may influence locked versus creeping behavior at subduction megathrusts. It will target portions of the plateau where the sedimentary cover is less than several hundred meters, well east of the deformation front, to avoid thick trench-fill sections near the Hikurangi Trough. We will also target expanded sections of the portions of incoming stratigraphy that correlate with where the plate boundary décollement is forming.
4.2 Episodic fluid flow driven by slow slip and its impact on gas hydrate systems on the Hikurangi margin
Bottom simulating reflectors (BSRs) observed at the Hikurangi subduction margin and their relationship to geothermal heat flow changes suggest that regional gas hydrate systems may be strongly influenced by episodic fluid flow processes. These processes may be driven by large strain transients that occur during episodic slow slip events. This proposal seeks to install subseafloor observatories to monitor pore pressure and temperature changes throughout the slow slip cycle. Genius plugs with osmotic samplers could undertake time-series sampling of fluids to evaluate changes in geochemistry with time. These observatories will enable evaluation of the impact of fluid pulsing on gas hydrate systems, and also quantify the degree of overpressure that builds up beneath hydrate systems during and between fluid pulsing, potentially driven by slow slip events. The latter could also play a role in submarine slope stability processes. Installation of a denser network of simple observatories will also enable more detailed spatiotemporal investigation of the distribution of offshore slow slip events, allowing many questions about shallow slow slip distribution and its impact on hydrogeology in the upper plate to be addressed.
4.3 Offshore freshwater resources in the Canterbury Basin
Results from IODP Expedition 317 in the Canterbury Basin showed a freshening signature at ∼ 50 m depth at Site U1353, while nearby Site U1354 showed near-seawater salinity. This transition from a freshwater-charged zone to a non-freshwater zone makes an interesting and well characterized target to investigate the dynamics of, and interactions between, freshwater and seawater subseafloor hydrological systems. Abundant regional site survey data exist in this area, including a recent voyage that acquired seismic and controlled source electromagnetic data, to allow investigation of the freshwater system beneath the offshore Canterbury Basin. A future drilling proposal will include active pumping tests and potentially an observatory component to look at transients in these systems. Such an effort should also be of great interest to the biological community as the communities of freshwater and saline systems will be very different. Evaluating the communities in the transition between these systems should provide insights into microbiology and nutrient availability.
4.4 Eruptive processes and transport in submarine volcanic environments along the Kermadec Ridge
Marine volcanic eruptive processes and underwater transport/deposition of volcanic material are poorly understood. In particular, the transport and depositional processes during submarine eruptions, and the behavior of pyroclastic flows as they transition from onshore to offshore environments is understudied. In some historical cases (such as the Krakatoa eruption), large tsunami have resulted from these processes, so understanding the underlying mechanisms of eruption-fed volcaniclastic transport into and under water also has important geohazard implications. Recent drilling in the Izu–Bonin–Mariana arc system uncovered 20–100 m thick eruption-fed units, but drilling on the flanks of submarine volcanoes is more suited to fully investigate these processes. The Kermadec Arc is an attractive location for such an effort, because (a) a number of submarine volcanoes have always been submarine and also have produced eruptions with significant volume, and (b) Macauley Island is an excellent locale to investigate pyroclastic transport and depositional processes into the sea, where arcuate sediment waves are observed on the order of 100 m high and 1 km long. Key questions include (1) what are the physics and processes behind submarine and coastal volcanic eruptions and subsequent deposition of their products, and (2) are the eruption products emplaced all at once, or do they occur in multiple episodes?
5.1 Did CO2 from geologic sources contribute to glacial–interglacial pCO2 variability, and cause the formation of seafloor pockmarks on the Chatham Rise, New Zealand?
After three decades of scientific effort there is no definitive answer to the question ”What Earth system processes were responsible for the systematic variations in atmospheric CO2 during each glacial cycle of the late Pleistocene?” Our proposal seeks to investigate and test the hypothesis that geologic reservoirs act as capacitors, storing large volumes of CO2 in marine sediments during glaciations and then leaking carbon to the ocean and atmosphere during glacial terminations. Recent discoveries have identified accumulations of both liquid and hydrate (∼ solid) CO2 in marine sediments at a variety of tectonic settings. These liquid and hydrate CO2 reservoirs can undergo phase changes as temperature and pressure changes during glacial–interglacial cycles, affecting the storage and leakage of carbon to the overlying ocean. Evidence supporting this hypothesis includes large Δ14C excursions in marine carbonates from the last glacial–interglacial transition that point to release of large quantities of 14C-dead carbon to the ocean. To date, the largest Δ14C excursions have been documented in the South Pacific on Chatham Rise and from the Galapagos margin. On Chatham Rise the Δ14C excursions coincided with the formation of large pockmarks. The pockmarks extend over an area of > 20 000 km2 and have been observed in seismic profiles in association with previous glacial terminations. The close temporal relationship between the Δ14C excursions and the formation of pockmarks points to a causal relationship whereby CO2 released from geologic reservoirs on Chatham Rise during the glacial–interglacial transition produced the pockmarks. The proposal sets forth a plan to investigate and test this hypothesis by obtaining and then studying sediment records from Chatham Rise that span each of the glacial cycles of the late Pleistocene.
5.2 Southeastern Chatham Rise margin: tectonics, dynamics, and paleoceanography
The southeastern continental margin of Chatham Rise is conjugate to the Amundsen Sea margin of West Antarctica. Deep crustal seismic, gravity, and magnetic data coupled with dredged samples from seamounts reveal a complex transition from continental to oceanic crust on both conjugate margin segments. In particular, the southeastern Chatham Terrace is underlain by a broad zone of thinned and fragmented transitional crust, presumably containing continental blocks separated by zones of oceanic crust. The nature of this type of transitional crust and the processes of its generation during Cretaceous rifting and breakup is poorly understood. The southern Chatham Rise is an ideal location to investigate crustal fragmentation during continental breakup by drilling into the different crustal zones, and could be combined with drilling into well-imaged sediment drifts to address hypotheses related to the development and evolution of southwestern Pacific Ocean circulation (e.g., Deep Western Boundary Current (DWBC) and ACC) during the Cenozoic.
5.3 Totten Glacier Cenozoic ice sheet evolution and sensitivity to past warming
Vulnerability of the East Antarctic Ice Sheet (EAIS) to climate change is uncertain. The low-lying, glacially sculpted Aurora Subglacial Basin (ASB; ∼ 3–5 m sea-level-equivalent ice) is a major marine-based East Antarctic catchment that drains ice from the Gamburtsev Mountains to the Sabrina Coast. The catchment consists of several over-deepened basins and hosts an active subglacial hydrological system, suggesting that regional ice may be susceptible to climate variability, particularly during warm climate intervals. New sediment records from the Sabrina Coast continental shelf will enable us to test fundamental hypotheses related to the existence of warm high southern latitude climates during the late Mesozoic and early Cenozoic, and evolution of the EAIS in the ASB from the Paleogene to the last deglaciation. The stratigraphic sequence also records variable meltwater influence, potentially critical for understanding catchment ice dynamics. Shelf records, though inherently discontinuous, offer advantages over deep-sea records, including the following: (1) direct records of ice margin fluctuations (e.g., lithologic changes, glacial erosion surfaces) and continental conditions (e.g., vegetation, temperature, hydrology), (2) shallow access to older strata due to tilting and glacial erosion of overlying strata, and (3) high sedimentation rates and shallow water depths, which favor carbonate macro- and microfossil (e.g., foraminifera, bivalves) preservation. Drilling the Sabrina Coast shelf will be technologically challenging. Proposed Sabrina Coast sites are located within a small polynya, which enhances ice risks and requires a mission specific platform. Drilling from a stable seabed drill or using ANDRILL-style riser drilling technology from an icebreaker will maximize recovery. This accessible archive of past Antarctic climate and ice sheet history will provide data to improve ice sheet and climate model boundary conditions and outputs. This type of data-model integration is required to better understand the response of Antarctica's ice sheets to continued anthropogenic warming.
5.4 Sabrina Coast slope deposits
This project aims to obtain high-latitude paleoclimate records from the Miocene to Pleistocene of ice sheet and ocean interactions at the East Antarctic margin to understand the history of Totten Glacier mobility and melting. It will obtain more continuous records of the oceanic drivers and responses to East Antarctic Ice Sheet variability than drilling on the continental shelf. It will also seek to obtain pre-Miocene records during past greenhouse climates, and correlation to continental shelf records in the Totten Glacier region in the proposal above (Sect. 5.3). Extensive seismic lines exist across the area with more than 28 crossing lines for selection of many potential drill sites. Turbidite overbank deposits are proposed as targets, as these were demonstrated during IODP Expedition 318 (Wilkes Land margin of East Antarctica) to provide high-resolution continuous archives of glacially-influenced sedimentation. Critically, such archives have proven valuable in identifying ice-sheet retreat events and characterizing these in the context of associated oceanographic change. Experience from an R/V Investigator voyage during January to March 2017 pointed to favorable weather conditions for coring in this area using standard JOIDES Resolution riserless drilling.
5.5 Southeastern Indian Ocean deep circulation and sediment drift history, basement depth, and mantle chemistry anomalies
The Southern Ocean encircles a highly dynamic glaciated Antarctic margin, and accommodates the amalgamation of several major water masses. Changes in the vigor of this top-to-bottom current would have significant implications for the exchange of heat between the Pacific, Indian, and Atlantic ocean basins, and may have consequences for the ventilation and primary productivity of the Southern Ocean. Contourite drifts are rapidly-deposited signatures of bottom current activity, and provide high-resolution records of paleoceanographic change. There are several lines of evidence suggesting that the Southeast Indian Ridge (SEIR) is covered extensively by a succession of Pleistocene to Pliocene-aged drifts. Long-term sedimentation rates exceed 5.5 cm kyr−1, and focusing factors suggest extensive sediment winnowing by lateral advection of bottom currents. Furthermore, drilling results from Deep Sea Drilling Project (DSDP) Site 265 indicate ”extremely high” sedimentation rates in the Quaternary. Recent ocean circulation numerical modeling also supports the accumulation of thick sediment drifts on the SEIR. The SEIR lies far from any terrigenous sources that could mask or otherwise contaminate any signals of bottom current intensity manifested within these sediment drifts. It is straightforward to combine the climate goals of such a proposal with petrological sampling of the Australian–Antarctic Discordance (AAD) and the eastern SEIR, aimed at testing alternative hypotheses about the origin of geochemical and depth anomalies along the SEIR (westward plume/asthenospheric flow along eastern SEIR towards the AAD versus mid-ocean ridge migration over an ancient slab burial ground). The two issues are connected in that anomalously elevated ridge segments act as potential obstacles along which contourites are deposited, while anomalously deep troughs and segments of the ridge may allow deep water to pass from one ridge flank to the other.
5.6 The Indian Ocean dipole and monsoon
The recovery of a sequence of Miocene to recent sediments from the eastern equatorial Indian Ocean will help resolve the history of the Indian Ocean dipole (IOD) on annual to tectonic timescales. The objectives of the drilling are to understand the following: (1) the evolution of sea surface temperatures (SSTs) in the eastern Indian Ocean since the Miocene, (2) the long-term relationship between eastern Indian Ocean SSTs and strengthening/weakening of the Indian monsoon, (3) the response of eastern Indian Ocean SSTs/IOD to atmospheric CO2 forcing, and (4) the influence of a constricted Indonesian Throughflow (ITF) gateway at ∼ 3–4 Ma on the IOD. We propose drilling a latitudinal transect at ∼ 5∘ S (∼ 90–110∘ E) to obtain longer timescale records as old as early Miocene, in order to understand the evolution of SSTs and the effect of ITF gateway closure on IOD. Furthermore, we propose drilling off the west coast of Sumatra, which will be helpful in obtaining high-resolution sediment cores to understand variations in the IOD at decadal to centennial timescales. This proposal will be amalgamated with an earlier plan, which aimed to understand Nicobar fan evolution, monsoon intensity and Himalayan uplift, and the stress state in oceanic crust and relationship to seismicity.
5.7 Future IODP drilling in northern Zealandia/Lord Howe Rise
Northern Zealandia and the Lord Howe Rise were drilled during IODP Expedition 371 using JOIDES Resolution from July to September 2017, and is planned to be drilled during a SEP/CIB-approved D/V Chikyu expedition that, subject to funding, is scheduled for the second half of 2020 (Complementary Project Proposal 871-CPP). New opportunities for drilling in northern Zealandia will undoubtedly emerge from the core and data collected during Expedition 371, which is investigating Eocene Tonga-Kermadec subduction initiation and evaluating whether a period of high-amplitude long-wavelength compression led to initiation of subduction or determine if alternative geodynamic models were involved. Moreover, Paleogene and Neogene sediments recovered during Expedition 371 are also constraining paleoceanographic changes caused by subduction initiation as well as tropical and polar climatic teleconnections and the transition from greenhouse to icehouse climate states. In the case of Proposal 871-CPP for deep riser drilling to investigate Lord Howe Rise crustal ribbon development, ocean biogeochemical cycles at high southern latitudes from the Cretaceous onwards, and the limits of life beneath the ocean floor, several proposed alternate sites may be suitable for riserless drilling using JOIDES Resolution.
New site survey data obtained in support of these recent and planned expeditions provide modern seismic coverage of the entire width of northern Zealandia from the Norfolk Ridge to the Tasman Sea oceanic basin. These data undoubtedly reveal a large number of additional drill sites, many of which have not yet been considered in detail. Another possible long-term objective in the region is to target seamounts and submerged plateaus within and to the north of northern Zealandia where drilling could address important geodynamic questions surrounding changes in Pacific Plate motion, and the connections among deep mantle plumes and large igneous provinces. It was agreed at the workshop that future plans for IODP drilling in northern Zealandia should be revisited in mid-2018 after results from Expedition 371 begin to emerge and the status of funding and logistics for Proposal 871-CPP is clearer.
5.8 Completing the Australian–Antarctic transect
The Australo–Antarctic rift system affords an opportunity to document lithosphere thinning history during continental breakup, and to understand the transition between rift and oceanic crust formation. The peridotite ridge in this region, representing the boundary between continental and oceanic crust, has risen high enough to be reached with riserless drilling. A second aim is to understand the timing, nature, and consequences of post-rift subsidence of the outer continental shelf of both the Australian and Antarctic margins. It is expected that post-rift subsidence was minimal, because continental migration was compensated for by formation of oceanic crust. Both margins should be completely independent in terms of subsidence history as soon as oceanic crust formation commences, but evidence from IODP Expedition 318 (Wilkes Land) suggests that the outer continental shelf of the Antarctic margin collapsed long after oceanic crust started forming in the rift system. Moreover, and surprisingly, seismic profiles along the conjugate Australian and Antarctic margins show considerable symmetry. However, on the Australian side, we lack recovered sedimentary records that allow dating of the sediments from the seaward limit of the continental margin. The region also has fundamental climate questions to address, including the deep-sea expression of Eocene–Oligocene glaciation and circum-Antarctic erosion, and the history of the development of the ACC and spatial migration of Southern Ocean frontal systems. It was proposed to develop a plan to drill a transect which connects the Otway–Ceduna basins (Australian margin) and the Antarctic margin.
6.1 Reflux brines: linking continental shelf hydrogeology to subseafloor microbiology
The role of mass transport in continental margin environments has historically been underappreciated. Recent oceanographic tracer studies indicate that discharge of saline groundwater from passive continental margins occurs at rates equal to, or exceeding, river discharge. This implies large-scale migration of saline groundwater through continental shelf sediments and is consistent with decades of research in carbonate diagenesis, where the importance of groundwater mass transport has long been recognized. Sea-level pumped reflux brines, formed by evaporation of seawater on the exposed shelf during sea-level minima, should be common in subtropical passive margin sequences, and may provide the missing mechanism to explain the large-scale dolomitization and mineralization processes observed throughout Earth's history. These shelf-scale hydrological systems may also support abundant deep microbial life on the upper shelf slope.
Results from ODP Leg 182 show that Great Australian Bight (GAB) likely contains an actively discharging reflux brine system. Two transects across the outer GAB margin were proposed to assess coupled groundwater flow, geochemical reactions and microbial metabolic processes. Results from ODP Leg 182 suggest that the brine-supported microbial ecosystem in the GAB thrives under hyper-alkaline and hyper-sulfidic conditions, which are profoundly distinct from most other known deep biosphere environments. The tantalizing possibility is that we will gain an unprecedented glimpse into the microbial and organic geochemical processes that are responsible for the formation of a large portion of the world's hydrocarbon resources, as well as determining the role of saline groundwater flow in carbonate diagenesis in continental margin environments.
6.2 The edge of the gyre: biological and oceanographic transitions from the South Pacific Gyre into the Southern Ocean through the Cenozoic
A transect of sites from the South Pacific Gyre into the Southern Ocean will record a north–south gradient of different biogeochemical and oceanographic regimes within oxic and suboxic sediments through the Cenozoic. Microbiological research will help to address questions honed from the results of IODP Expedition 329 to the South Pacific Gyre. One of its most southern sites (Site U1371) included a shift from pelagic clay sedimentation to siliceous accumulation at ∼ 8 Ma, and the microbial communities between these two lithological units are unique. Drilling additional sites on the southernmost edge of the South Pacific Gyre that focus on acquiring these types of depositional and biogeochemical transitions will allow the examination of how microbial ecosystems are established and respond to changing environments. This region is critically underexplored in ocean drilling, and numerous paleoceanographic questions could also be addressed in this understudied region, although further work is required to develop specific hypotheses to test.
The 97 scientists from 12 different countries gathered at the 2017 Australasian IODP Workshop in Sydney, tasked with planning scientific ocean drilling expeditions in the eastern Indian, southern, and southwestern Pacific oceans, emphasize the critical importance of geoscientific site characterization to the future success of IODP and its successors. Site characterization data, most importantly seismic reflection data, are essential for the identification of suitable primary and alternate drill sites in every full drilling proposal submitted to the IODP science support office, and are subsequently carefully considered by the program's science evaluation panel (SEP) and the three facility boards.
Without this type of information, the scientific exploration of the deep subseafloor and our understanding of its role in tectonic, climatic, oceanographic, biological, and geochemical processes in the Earth system cannot advance. Providing suitably capable vessels for that purpose is essential for the advancement of scientific ocean drilling as it addresses ever-evolving global scientific questions, particularly in underexplored parts of the world ocean like the Australasian region.
Accordingly, we emphasize that blue water research vessels with the necessary seismic reflection systems should continue to be available to researchers in all IODP member countries under reasonable fiscal conditions, and with suitable advance (national and international) planning mechanisms.
No data sets were used in this article.
The authors declare that they have no conflict of interest.
The organizers gratefully acknowledge generous and critically important
funding for participants' travel to the workshop. Funding came from the
Australian and New Zealand IODP Consortium (ANZIC), the US Science Support
Program (USSSP), the Magellan-Plus Workshop Program of the European
Consortium for Ocean Research Drilling (ECORD), the Japan Drilling Earth
Consortium (J-DESC), the Japan Agency for Marine-Earth Science and
Technology (JAMSTEC), IODP-India, and the home institutions of numerous
scientists. The University of Sydney assisted us in providing facilities for
the workshop.
Edited by: Jan Behrmann
Reviewed by: Antony Morris and one anonymous referee
- Abstract
- Introduction
- Large igneous provinces and associated paleoceanography
- Subduction zones
- New Zealand region: subduction inputs on Hikurangi Plateau, Hikurangi margin episodic fluid flow, Canterbury Basin fresh water, plus eruptive processes on Kermadec Ridge
- Conjugate margins and climate
- Biosphere
- Consensus statement regarding the critical importance of site characterization data for IODP scientific drilling proposals
- Data availability
- Competing interests
- Acknowledgements
- Abstract
- Introduction
- Large igneous provinces and associated paleoceanography
- Subduction zones
- New Zealand region: subduction inputs on Hikurangi Plateau, Hikurangi margin episodic fluid flow, Canterbury Basin fresh water, plus eruptive processes on Kermadec Ridge
- Conjugate margins and climate
- Biosphere
- Consensus statement regarding the critical importance of site characterization data for IODP scientific drilling proposals
- Data availability
- Competing interests
- Acknowledgements