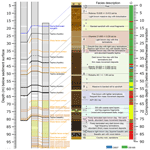
Stratigraphy and sedimentology of the Orakei maar lake sediment sequence (Auckland Volcanic Field, New Zealand)
Paul C. Augustinus
Global paleo-climate reconstructions are largely based on observations from the Northern Hemisphere despite increasing recognition of the importance of the Southern Hemisphere mid-latitudes for understanding the drivers of the global climate system. Unfortunately, the required complete and high-resolution terrestrial records from the Southern Hemisphere mid-latitudes are few. However, the maar lakes in the Auckland Volcanic Field (AVF), New Zealand, are crucial in this regard as they form outstanding depositional basins due to their small surface-to-depth ratio, restricted catchment, and absence of ice cover since their formation, hence ensuring continuous sedimentation with anoxic bottom water. Significantly, the estimated age of the AVF of ca. 250 ka may allow development of a continuous sediment record spanning the last two glacial cycles. The Orakei maar lake sediment sequence examined in this study spans the Last Glacial Cycle (ca. 126 to ca. 9.5 ka cal BP) from the phreatomagmatic eruption to the crater rim breach due to post-glacial sea-level rise. Two overlapping cores of >100 m sediment were retrieved and combined to develop a complete composite stratigraphy that is presently undergoing a wide range of multi-proxy analyses.
- Article
(3834 KB) - Full-text XML
-
Supplement
(341 KB) - BibTeX
- EndNote
Lake sediments are important archives for Quaternary paleo-environmental and paleo-climatic reconstruction, particularly when sedimentation has been continuous and sedimentation rates high, as they offer a variety of high-resolution proxy records of environmental change. In the context of the location of New Zealand in the Southern Hemisphere mid-latitudes, lakes act as important recorders of past climate in the south-western Pacific (Alloway et al., 2007). Furthermore, Auckland is situated at an ecological and climatic boundary between the subtropical north and the south of New Zealand influenced by subpolar climatic and oceanographic systems (Augustinus, 2007). Consequently, the up to 250 ka duration and high-resolution laminated sediment cores retrieved from maar lakes in the Auckland Volcanic Field (AVF) constitute ideal recorders of past regional climatic variability over the last two glacial cycles.
Due to their low diameter-to-depth ratio and limited catchment area (dominated by the lake itself), allochthonous sedimentation is a minor component of the infill, so that maar lake sediment is largely a direct archive of regional climatic signals (e.g. Augustinus et al., 2011; Brauer et al., 1999; Horrocks et al., 2005; Sandiford et al., 2003; Zolitschka et al., 2013). AVF maar lakes also preserve complete archives of eruptions of the local basaltic volcanoes as well as rhyolitic and andesitic volcanic systems situated in the Taupo Volcanic Zone (TVZ) and Egmont Volcano (Fig. 1) to the south of Auckland (Hopkins et al., 2015; Molloy et al., 2009; Shane, 2005; Shane and Hoverd, 2002). This is of importance as the City of Auckland, with >1.5 million inhabitants, is situated on a potentially active volcanic field for which eruption frequencies cannot be derived from the historical record (Edbrooke et al., 2003; Molloy et al., 2009; Newnham et al., 1999). Many basaltic tephra layers have been recorded in the AVF maar sediment cores, but age estimates rely on sedimentation rate extrapolation to and correlation with the source volcanoes, which mostly have not been dated unambiguously (Hopkins et al., 2015, 2017; Leonard et al., 2017; Lindsay et al., 2011; Molloy et al., 2009). Here, we focus on the Orakei maar paleo-lake, which contains a finely laminated sediment sequence with high sedimentation rates (average ∼0.7 mm yr−1) possibly spanning the Eemian (MIS 5e) to the earliest Holocene. After an initial coring campaign in 2007, which established the potential of the Orakei maar record (Hopkins et al., 2015; Molloy et al., 2009), two overlapping cores were recovered in 2016 that reached the primary volcanic ejecta at the base of the crater at ca. 105 m depth below the sediment–water interface. Emphasis in the present study lies in establishing a detailed composite stratigraphy by aligning the overlapping sediment cores collected.
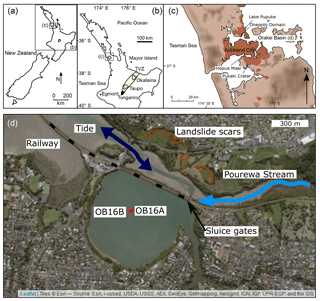
Figure 1Map of the Orakei maar study area. (a) New Zealand with insets (b) and (c) marked. (b) New Zealand's North Island with Auckland (inset c) and the major volcanic source centres marked. (c) Auckland area showing the position of Orakei Basin. Red shading in populated areas; dark shading shows increased elevation. (d) Satellite image (ESRI world imagery) show the coring locations with red dots and other important features of the surroundings of the maar.
Many sediment records rely on one master record stitched together from two or more cores from a sedimentary sequence, although it is often not explained how these master records are derived. Nakagawa et al. (2012) summarised an incomplete early sediment sequence retrieved from Lake Suigetsu (Japan) resulting in potentially erroneous interpretations. Furthermore, they reported details of aligned sediment core sections from the Lake Suigetsu (Japan) sequence drilled in 2006, which largely relied on visual identification of marker layers (i.e. tephra and flood layers), as well as intervals of lower confidence in the alignment of the sediment cores without abundant visual markers. A recent approach using core-scanning X-ray fluorescence (XRF) by Mischke et al. (2017) aligned two cores using visually distinct features in the down-core elemental variation, but also noted the need to delete a number of “second order markers” to avoid conflicting and likely incorrect stratigraphic ordering.
Here we (1) provide details of the approach used for robust construction of a composite stratigraphy from two (or more) overlapping cores based on visual markers, and, if the latter are absent, guided by μ-XRF-based geochemical variability; and (2) introduce and describe the Orakei maar paleo-lake in a lithostratigraphic framework. In so doing we highlight the outstanding potential of the sequence for paleo-climatic reconstructions from an under-studied region of crucial importance to understanding the behaviour of the global climate system over the Last Glacial Cycle.
Auckland is located in the northern part of the North Island of New Zealand (Fig. 1a). The coring site, Orakei Basin, is a tidal lagoon with artificially controlled inflow and outflow to maintain water levels for water sports and allow regular flushing of pollutants. Subsequent to the highly explosive maar-forming phreatomagmatic eruption, a lake filled the crater until ca. 9.5 ka cal BP, when post-glacial sea-level rise breached the crater rim and connected the lake to the sea, allowing mass influx of tidal mud (Hayward et al., 2008). The modern surroundings of Orakei Basin are characterised by residential buildings to its south and the crater rim collapse identifiable from active landslides (Fig. 1d). Erosion and collapse of the steep crater walls and successive crater widening have led to repeated pulses of recycled eruptive material (juvenile clast and accidental ejecta/country rock) into the basin (Németh et al., 2012). Figure 1d shows the location of the two cores retrieved in 2016. No GPS position was recorded for the core retrieved in 2007, but the position was noted as near the centre of the basin. No shallow seismic survey of the sediment strata below the sediment–water interface has been undertaken. Hence, lateral extent and distribution of strata remain speculative.
3.1 Coring
Initial coring of Orakei Basin was undertaken from a floating barge in 2007 using a rotating barrel system, with a single core that penetrated to 81 m depth below the water–sediment interface. A further set of two overlapping cores with 50 cm vertical offset between them was collected in 2016 from a barge using wireline drilling and core collection in 1 m long sections. The lateral offset between the OB16A and OB16B core was 8 m.
3.2 Core description and visual facies identification
Split-core surfaces were described in detail for visually distinct changes in composition, qualitative grain size (clay, silt, very fine to coarse sand), colour (Munsell Color, 1975), thickness and shape of laminations, signs of bioturbation (burrows), (coring-induced and natural) disturbance of laminations and sedimentary features, presence of macrofossils (pieces of wood/bark and small twigs), and tephra layers. Each facies unit contains sediment of similar colour, composition, and laminae structure (or absence of laminations). Subunits differentiate between intervals of smaller differences, i.e. a change in lamination thickness or a slight colour change with overall similar sediment type and appearance. Most facies units have sharp contacts and are hence easily identified.
3.3 μ-XRF core scanning and magnetic susceptibility
The Orakei sediment cores were scanned using an Itrax μ-XRF core scanner (Cox Analytical Systems (Gothenburg, Sweden) at the School of Environment, University of Auckland, New Zealand. μ-XRF core scanning records down-core elemental variation in the range Al to U (on the Mo X-ray tube) rapidly, non-destructively, and with very little sample preparation (Croudace et al., 2006; Croudace and Rothwell, 2015). Here, μ-XRF data were acquired for all cores with the Mo X-ray tube (at 30 kv, 55 mA) at 1 mm spatial resolution and 10 s/step exposure time. High-resolution optical images (at 47 µm) and radiographic images (at 60 kV, 50 mA and 1 mm resolution) were recorded during the same scanning process. Magnetic susceptibility was acquired automatically by the same Itrax core scanner at 5 mm resolution for all cores with a Bartington Magnetic Susceptibility MS2e surface scanning sensor.
3.4 Establishing the composite stratigraphy
The two cores retrieved in 2016 were drilled with at least 50 cm vertical offset to allow for overlaps between both cores around core breaks. The alignment of these overlapping sections is straightforward by visual means in the finely laminated sediment sections of the core. However, it is more difficult to establish a composite stratigraphy in the coarser sediment where clear marker layers are often absent. Consequently, magnetic susceptibility and μ-XRF data were used to aid the correlation of the overlapping cores as described below.
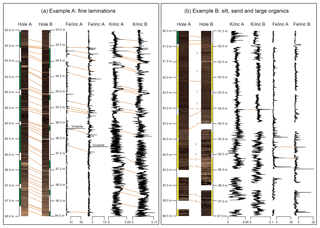
Figure 2Examples of core photos from the Orakei maar paleo-lake record and their correlation. (a) Laminated facies easily correlated using visual marker layers. Down-core μ-XRF variation can be used for correlation, but does not offer additional markers. Green bands along the depth axis indicate the record of higher quality, which is adopted for the composite μ-XRF and MS record. (b) Facies comprised of silt, sand, and large subfossil wood fragments which are very difficult to correlate unambiguously. Visual correlation may be incorrect in large sections, but cannot be improved easily with μ-XRF variation either. Hence, yellow bands alongside the depth axis indicate unsure correlation, which is carried forward in the master record.
Visually prominent marker layers allowed most of the correlation using tephra layers (Table S2 in the Supplement), thick mass movement deposits, and major sharp facies contacts (i.e. onset of laminations, strong changes in grain size or colour) as outlined in Figs. 2 and 3. Finely laminated sediment was aligned on a sub-centimetre scale visually as highlighted by the high-resolution images in Fig. 2 (left). Coarse sediment showing no clear features along which to align the cores is shown in Fig. 2 (right). To obtain a complete composite stratigraphy, these intervals are correlated with reduced certainty (marked yellow in Fig. 3). These intervals constitute ca. 15 % of the entire sediment sequence and are confined by sharp upper and lower contacts. K and Fe (normalised to incoherent scatter (inc)) have been identified as showing the highest potential for cross-core correlation and have been used to identify chemical changes where visual markers are absent. The feasibility of this approach is underlined by the alignment of the finely laminated sediment where XRF-based correlation is in agreement with visual correlation as shown in Fig. 2 (left). Prominent minima or maxima in the geochemical XRF curve (i.e. K/inc) occur at sediment horizons different from sediment below and/or above, such as event layers or facies changes. It is reasonable to assume that these horizons are recorded in all sediment cores of the same depositional basin, which underlines their potential as marker layers. In this way, the down-core elemental variability has been used as an additional aid to establishing an overlapping composite stratigraphy. Where no other constraint on correlation could be established, it was guided by the common offset of the A-core usually showing distinct features ∼1 m higher than the B-core (note the offset depth scales in Fig. 2 for visualisation purposes). Horizons of instantaneous deposition such as tephra layers and mass movement deposits were removed on the event-corrected depth scale (ECD).
4.1 Lithostratigraphic units
Figure 3 summarises the 16 major lithostratigraphic units (numbers) with 31 subunits (letters). Their differentiation is driven by visual changes in colour, grain size, dominant structure, and lamination thickness. Facies descriptions of the units are provided in Table S1 in the Supplement and are shown in Fig. 3, with example core photos in Fig. 4.
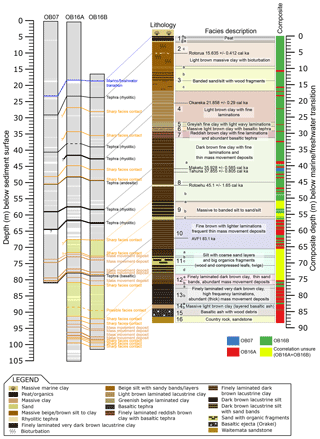
Figure 3Orakei maar paleo-lake composite stratigraphy, lithological description, and rhyolitic marker tephra layers with ages (tephra layers from Peti et al., 2019).
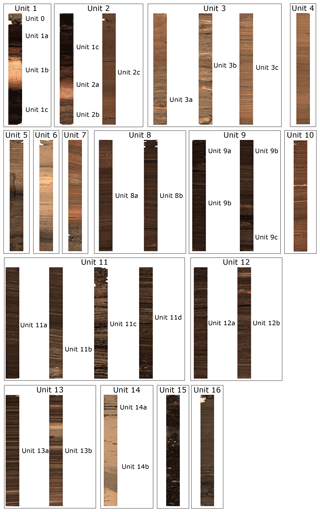
Figure 4Example core photographs of the 16 facies units and subunits. Each core photo graphed is ∼1 m long.
4.2 Preliminary age model
The dated rhyolitic tephra marker layers and one radiocarbon date near the top of the sequence from the 2007 core published in Hayward et al. (2008) (NZA28865: 9512±19 cal ka BP, calibrated with SHCal13; Hogg et al., 2013) allow for the construction of a preliminary, simplified age model (Fig. 5). The finely laminated sediment between the Tahuna and Rotoehu tephra is largely representative for most of the Orakei sediment sequence below 40 m (ECD). The thick sand bands of facies units 9 and 11 have been removed on the ECD, explaining the difference of 4.83 m between Figs. 3 and 5. The sediment between those bands is mostly laminated silt, and we assume a similar sedimentation rate both above and below for this preliminary age estimate for the core base (Fig. 5). Extrapolation of the sedimentation rate between the Tahuna and Rotoehu tephras (2300 yr m−1) to the base of the sequence (hence, eruption age of Orakei crater) produces an age of ca. 123.6 ka BP (Fig. 5, age c). Using the same approach, the age for basaltic tephra layer AVF1 is estimated to be ca. 80.4 ka BP, only slightly younger than the 83.1 ka BP estimated by Molloy et al. (2009) and well within typical errors of tephra ages pre-21 ka BP of ±1–2 ka (Molloy et al., 2009). Hopkins et al. (2017; Fig. 5) inferred an older age for AVF1 of 106.17±4.3 ka BP together with a basal age of 126.15±3.32 ka BP. Although the age estimate of the base of the sequence (i.e. the Orakei eruption, correlated with the AVFa horizon in Hopkins et al., 2017) agrees very well with our estimates, the AVF1 age appears >20 kyr older compared to the estimate from the Orakei sediment accumulation rates (Fig. 5). This age–depth relationship would result in a low sedimentation rate between Rotoehu and AVF1 and a marked increase between AVF1 and the sequence base. Although it is possible that very thin mass movement deposits have not been recognised which could cause this higher sedimentation rate, the same would be true for facies units 8 and 10 above the AVF1 layer (Fig. 3). More rapid infilling of the crater could be associated with facies units 9 and 11 despite removal of the event layers. On the other hand, a slower observed sedimentation rate could also be caused by periods of non-deposition during significantly lower lake levels. Sharp facies contacts may indicate erosion and non-deposition. However, we consider this to represent only short time durations since the clay and silt intervals between the sand bands in facies units 9 and 11 show laminations indicating continuous sedimentation under deep-lake conditions (Fig. 3). Consequently, the inferred near-constant sedimentation rate model is preferred here. This model suggests that the Orakei eruption occurred just after Termination II (ca. 130 ka BP; Lisiecki and Raymo, 2005) early in the onset of the Last Interglacial (MIS 5e). Therefore, we infer that the Orakei sediment sequence spans most of the Last Glacial Cycle. However, these are necessarily crude estimates based on linear interpolation and extrapolation, and they are being refined (see Sect. 5).
A high-resolution multi-method chronology is currently being established for the Orakei sediment sequence based on radiocarbon dating, tephrochronology, post-IR IRSL (luminescence) dating, relative paleo-intensity variation of the Earth's magnetic field, and 10Be cosmogenic nuclide flux into the lake. Coeval with this work, pollen and chironomid analyses are underway to enable detailed reconstruction of changes in the paleo-vegetation and climatic conditions. Itrax μ-XRF core scanning data alongside traditional measurements of loss-on-ignition, carbon and nitrogen content, as well as stable oxygen and carbon isotopes, will be combined to reconstruct the climatic development of the area over the Last Glacial Cycle. Micro-facies studies on resin-impregnated sediment slabs and large-scale thin sections will allow identification of abrupt climate shifts and events as well as identification of changes in seasonality recorded in the Orakei composite sediment sequence.
The Orakei maar paleo-lake sequence is expected to do the following.
-
Constrain the frequency and magnitude of eruptions of the Auckland Volcanic Field through provision of better age estimates for past basaltic eruptions.
-
Constrain the nature, drivers, magnitude, and timing of climatic changes in this part of the south-western Pacific. In addition, accurate and precise dating of the age markers and correlation with cosmogenic nuclide production variation (10Be) may enable identification of climate leads and lags between the Northern and Southern Hemisphere mid-latitudes, including European maar lake and polar ice core records.
-
Understand the role of the westerly winds in climate change on a millennial to sub-decadal timescale in the south-western Pacific over the Last Glacial Cycle, with thick laminae likely reflecting a response to strong south-westerly winds with increased precipitation over the region depositing wind-blown dust and, hence, controlling biological productivity in the lake.
Even the most detailed observations and most sophisticated coring techniques cannot produce a perfect sediment core composite stratigraphy. In the case of finely laminated sediment and no to little coring disturbance, it is relatively straightforward to align overlapping cores along prominent sedimentary features, such as mass movement deposits, unique alternations of laminations, sharp facies contacts, or tephra layers. In the case of coarse sand and macroscopic organic detritus (e.g. facies units 9 and 11), core alignments prove to be more challenging. Despite the close proximity of the two coring positions in Orakei maar (Fig. 1, 10 m distance), the uneven distribution of mass movement deposits/slumps at the lake bottom results in small differences of the sediment stratigraphy that reduce confidence in the development of the composite stratigraphy over these intervals. While it is important to be aware of this source of uncertainty, the affected interval will reduce in relative thickness when the record is viewed against a timescale as opposed to a depth scale (metres below the marine water–freshwater transition).
Overall, the Orakei maar paleo-lake record constitutes an exceptional, continuous, long, high-resolution sedimentary record that captures environmental and climatic changes in the northern North Island of New Zealand over the Last Glacial Cycle. The history of the lake commenced with the maar-forming phreatomagmatic eruption, through various stages of changing deep lacustrine conditions, minor mass movement events associated with erosion/collapse of the crater rim, and deposition of distal rhyolitic and locally derived basaltic tephra layers. The deep lake phase was terminated by fluvial sediment influx at ca. 20.3 cal ka BP (onset of facies unit 3) and peat accumulation to infill the basin before an episodic breach of the crater rim associated with rising post-glacial sea level in the early Holocene drove rapid marine sediment influx to create the modern tidal basin. Correlation of the Orakei maar lake sediment sequence with those developed from Pupuke and Onepoto maars (Fig. 1) will enable development of an AVF master stratigraphy and robust reconstruction of environmental and climatic conditions spanning the last two glacial cycles for the first time from subtropical northern New Zealand.
Preliminary data presented in this progress report are not publicly available as they are still being evaluated and expanded. Upon completion, all data will be made available when scientific papers and reports are published.
The supplement related to this article is available online at: https://doi.org/10.5194/sd-25-47-2019-supplement.
PCA leads the AVF maar project, secured funding, and is involved in all aspects of the work. LP conducted the core descriptions, Itrax μ-XRF core scans, and development of the composite stratigraphy. LP wrote the original version of this report with refinements from PCA.
The authors declare that they have no conflict of interest.
The drilling investigation team included Paul Augustinus, Jan Lindsay, Phil Shane, Elaine Smid (University of Auckland), Jenni Hopkins and Graham Leonard (GNS Science), Valerie van den Bos (Victoria University of Wellington), Tim Shanahan and Natalia Piatrunia (University of Texas at Austin), and Frank Sirocko (University of Mainz). The project was funded by DeVORA (funded by New Zealand's Earthquake Commission and the Auckland Council), and a grant from the Royal Society of New Zealand Marsden Fund (UOA1415 to Paul C. Augustinus). Leonie Peti thanks Patricia Gadd (ANSTO, Australia) and Per Engström (Cox Analytical, Sweden) for outstanding help with Itrax data. We thank Bernd Zolitschka and David Lowe for thoughtful comments and improvements on the manuscript and Ulrich Harms for efficient editorial handling.
This paper was edited by Ulrich Harms and reviewed by David Lowe and Bernd Zolitschka.
Alloway, B. V., Lowe, D. J., Barrell, D. J. A., Newnham, R. M., Almond, P. C., Augustinus, P. C., Bertler, N. A. N., Carter, L., Litchfield, N. J., McGlone, M. S., Shulmeister, J., Vandergoes, M. J., Williams, P. W., and NZ-INTIMATE members: Towards a Climate Event Stratigraphy for New Zealand over the past 30 000 years (NZ-INTIMATE project), J. Quaternary Sci., 22, 9–35, https://doi.org/10.1002/Jqs.1079, 2007.
Augustinus, P.: NZ-Maars: Extracting high resolution paleoclimate records from maar crater lakes, Auckland, New Zealand, PAGES News, 15, 18–20, 2007.
Augustinus, P., D'Costa, D., Deng, Y., Hagg, J., and Shane, P.: A multi-proxy record of changing environments from ca. 30 000 to 9000 cal. a BP: Onepoto maar palaeolake, Auckland, New Zealand, J. Quaternary Sci., 26, 389–401, https://doi.org/10.1002/jqs.1463, 2011.
Brauer, A., Endres, C., and Negendank, J. F. W.: Lateglacial calendar year chronology based on annually laminated sediments from Lake Meerfelder Maar, Germany, Quaternary Int., 61, 17–25, https://doi.org/10.1016/S1040-6182(99)00014-2, 1999.
Croudace, I. W. and Rothwell, R. G. (Eds.): Micro-XRF Studies of Sediment Cores: Applications of a non-destructive tool for the environmental sciences, Springer, DPER Series 17, Dordrecht, 656 pp., 2015.
Croudace, I. W., Rindby, A., and Rothwell, R. G.: ITRAX: description and evaluation of a new multi-function X-ray core scanner, Geol. Soc. Lond. Spec. Publ., 267, 51–63, https://doi.org/10.1144/GSL.SP.2006.267.01.04, 2006.
Edbrooke, S. W., Mazengarb, C., and Stephenson, W.: Geology and geological hazards of the Auckland urban area, New Zealand, Quaternary Int., 103, 3–21, https://doi.org/10.1016/S1040-6182(02)00129-5, 2003.
Hayward, B. W., Morley, M. S., Sabaa, A. T., Grenfell, H. R., Daymond-King, R., Molloy, C., Shane, P. A., and Augustinus, P. A.: Fossil Record of the Post-Glacial Marine Breaching of Auckland's Volcanic Maar Craters, Rec. Auckl. Museum, 45, 79–99, 2008.
Hogg, A., Hua, Q., Blackwell, P. G., Niu, M., Buck, C. E., Guilderson, T. P., Heaton, T. J., Palmer, J. G., Reimer, P. J., Reimer, R. W., Turney, C. S. M., and Zimmerman, S. R. H.: SHCal13 Southern Hemisphere Calibration, 0–50,000 Years cal BP, Radiocarbon, 55, 1889–1903, https://doi.org/10.2458/azu_js_rc.55.16783, 2013.
Hopkins, J. L., Millet, M. A., Timm, C., Wilson, C. J. N., Leonard, G. S., Palin, J. M., and Neil, H.: Tools and techniques for developing tephra stratigraphies in lake cores: A case study from the basaltic Auckland Volcanic Field, New Zealand, Quaternary Sci. Rev., 123, 58–75, https://doi.org/10.1016/j.quascirev.2015.06.014, 2015.
Hopkins, J. L., Wilson, C. J. N., Millet, M. A., Leonard, G. S., Timm, C., McGee, L. E., Smith, I. E. M., and Smith, E. G. C.: Multi-criteria correlation of tephra deposits to source centres applied in the Auckland Volcanic Field, New Zealand, B. Volcanol., 79, 55, https://doi.org/10.1007/s00445-017-1131-y, 2017.
Horrocks, M., Augustinus, P., Deng, Y., Shane, P., and Andersson, S.: Holocene vegetation, environment, and tephra recorded from Lake Pupuke, Auckland, New Zealand, New Zeal. J. Geol. Geop., 48, 85–94, https://doi.org/10.1080/00288306.2005.9515100, 2005.
Leonard, G. S., Calvert, A. T., Hopkins, J. L., Wilson, C. J. N., Smid, E. R., Lindsay, J. M., and Champion, D. E.: High-precision 40Ar∕39Ar dating of Quaternary basalts from Auckland Volcanic Field, New Zealand, with implications for eruption rates and paleomagnetic correlations, J. Volcanol. Geoth. Res., 343, 60–74, https://doi.org/10.1016/j.jvolgeores.2017.05.033, 2017.
Lindsay, J., Leonard, G., Smid, E., and Hayward, B.: Age of the Auckland Volcanic Field: a review of existing data, New Zeal. J. Geol. Geop., 54, 379–401, https://doi.org/10.1080/00288306.2011.595805, 2011.
Lisiecki, L. E. and Raymo, M. E.: A Pliocene-Pleistocene stack of 57 globally distributed benthic δ18O records, Paleoceanography, 20, 1–17, https://doi.org/10.1029/2004PA001071, 2005.
Mischke, S., Lai, Z., Aichner, B., Heinecke, L., Mahmoudov, Z., Kuessner, M., and Herzschuh, U.: Quaternary Geochronology Radiocarbon and optically stimulated luminescence dating of sediments from Lake Karakul, Tajikistan, Quat. Geochronol., 41, 51–61, https://doi.org/10.1016/j.quageo.2017.05.008, 2017.
Molloy, C., Shane, P., and Augustinus, P.: Eruption recurrence rates in a basaltic volcanic field based on tephralayers in maar sediments: Implications for hazards in the Auckland volcanic field, Bull. Geol. Soc. Am., 121, 1666–1677, https://doi.org/10.1130/B26447.1, 2009.
Munsell Color: Munsell soil color charts, Munsell Color, Baltimore, Md., 1975.
Nakagawa, T., Gotanda, K., Haraguchi, T., Danhara, T., Yonenobu, H., Brauer, A., Yokoyama, Y., Tada, R., Takemura, K., Staff, R. A., Payne, R., Bronk Ramsey, C., Bryant, C., Brock, F., Schlolaut, G., Marshall, M., Tarasov, P., and Lamb, H.: SG06, a fully continuous and varved sediment core from Lake Suigetsu, Japan: Stratigraphy and potential for improving the radiocarbon calibration model and understanding of late Quaternary climate changes, Quaternary Sci. Rev., 36, 164–176, https://doi.org/10.1016/j.quascirev.2010.12.013, 2012.
Németh, K., Cronin, S. J., Smith, I. E. M., and Agustin Flores, J.: Amplified hazard of small-volume monogenetic eruptions due to environmental controls, Orakei Basin, Auckland Volcanic Field, New Zealand, B. Volcanol., 74, 2121–2137, https://doi.org/10.1007/s00445-012-0653-6, 2012.
Newnham, R. M., Lowe, D. J., and Alloway, B. V.: Volcanic hazards in Auckland, New Zealand: a preliminary assessment of the threat posed by central North Island silicic volcanism based on the Quaternary tephrostratigraphical record, Volcanoes Quat., 161, 27–45, https://doi.org/10.1144/GSL.SP.1999.161.01.04, 1999.
Peti, L., et al.: Reliability and repeatability of bulk μ-XRF measurements of rhyolitic tephra for their rapid and non-destructive identification, J. Quaternary Sci., in review, 2019.
Sandiford, A., Newnham, R., Alloway, B., and Ogden, J.: A 28 000–7600 cal yr BP pollen record of vegetation and climate change from Pukaki Crater, northern New Zealand, Palaeogeogr. Palaeocl., 201, 235–247, https://doi.org/10.1016/S0031-0182(03)00611-4, 2003.
Shane, P.: Towards a comprehensive distal andesitic tephrostratigraphic framework for New Zealand based on eruptions from Egmont volcano, J. Quaternary Sci., 20, 45–57, https://doi.org/10.1002/jqs.897, 2005.
Shane, P. and Hoverd, J.: Distal record of multi-sourced tephra in Onepoto Basin, Auckland, New Zealand: Implications for volcanic chronology, frequency and hazards, B. Volcanol., 64, 441–454, https://doi.org/10.1007/s00445-002-0217-2, 2002.
Zolitschka, B., Anselmetti, F., Ariztegui, D., Corbella, H., Francus, P., Lücke, A., Maidana, N. I., Ohlendorf, C., Schäbitz, F., and Wastegård, S.: Environment and climate of the last 51,000 years – new insights from the Potrok Aike maar lake Sediment Archive Drilling prOject (PASADO), Quaternary Sci. Rev., 71, 1–12, https://doi.org/10.1016/j.quascirev.2012.11.024, 2013.