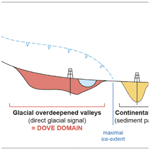
Drilling Overdeepened Alpine Valleys (ICDP-DOVE): quantifying the age, extent, and environmental impact of Alpine glaciations
Flavio S. Anselmetti
Milos Bavec
Christian Crouzet
Markus Fiebig
Gerald Gabriel
Frank Preusser
Cesare Ravazzi
The sedimentary infill of glacially overdeepened valleys (i.e., structures eroded below the fluvial base level) is an excellent but yet underexplored archive with regard to the age, extent, and nature of past glaciations. The ICDP project DOVE (Drilling Overdeepened Alpine Valleys) Phase 1 investigates a series of drill cores from glacially overdeepened troughs at several locations along the northern front of the Alps. All sites will be investigated with regard to several aspects of environmental dynamics during the Quaternary, with focus on the glaciation, vegetation, and landscape history. Geophysical methods (e.g., seismic surveys), for example, will explore the geometry of overdeepened structures to better understand the process of overdeepening. Sedimentological analyses combined with downhole logging, analysis of biological remains, and state-of-the-art geochronological methods, will enable us to reconstruct the erosion and sedimentation history of the overdeepened troughs. This approach is expected to yield significant novel data quantifying the extent and timing of Middle and Late Pleistocene glaciations of the Alps. In a first phase, two sites were drilled in late 2021 into filled overdeepenings below the paleolobe of the Rhine Glacier, and both recovered a trough filling composed of multiphase glacial sequences. Fully cored Hole 5068_1_C reached a depth of 165 m and recovered 10 m molasse bedrock at the base. This hole will be used together with two flush holes (5068_1_A, 5068_1_B) for further geophysical cross-well experiments. Site 5068_2 reached a depth of 255 m and bottomed out near the soft rock–bedrock contact. These two sites are complemented by three legacy drill sites that previously recovered filled overdeepenings below the more eastern Alpine Isar-Loisach, Salzach, and Traun paleoglacier lobes (5068_3, 5068_4, 5068_5). All analysis and interpretations of this DOVE Phase 1 will eventually lay the ground for an upcoming Phase 2 that will complete the pan-Alpine approach. This follow-up phase will investigate overdeepenings formerly occupied by paleoglacier lobes from the western and southern Alpine margins through drilling sites in France, Italy, and Slovenia. Available geological information and infrastructure make the Alps an ideal area to study overdeepened structures; however, the expected results of this study will not be restricted to the Alps. Such features are also known from other formerly glaciated mountain ranges, which are less studied than the Alps and more problematic with regards to drilling logistics. The results of this study will serve as textbook concepts to understand a full range of geological processes relevant to formerly glaciated areas all over our planet.
Overdeepened valleys and basins are commonly found below the present landscape surface in areas formerly affected by glaciations. As overdeepened structures reach below the fluvial base level (Fig. 1, left), they are interpreted as being of glacial origin, presumably formed by pressurized subglacial meltwater and ice-contact processes (e.g., Alley et al., 2019; Huuse and Lykke-Andersen, 2000). Limited awareness of subglacial erosion features historically posed severe challenges such as the accident in 1908 in the Bernese Alps (Switzerland) when a tunnel engineering project unexpectedly drilled into unconsolidated Quaternary sediments full of pressurized groundwater in an unknown overdeepening, causing 25 casualties (Fig. 1, right). In the Alps, overdeepened features are mainly associated with tectonic structures, weak lithologies, and/or Quaternary ice confluence and diffluence situations. Overdeepenings occur in buried elongated valleys, mainly oriented parallel to former ice flow, and in basins in the ablation area of glaciers (Preusser et al., 2010). The role of tectonics is still to be assessed, as regional differential uplift, fold growth, and active thrusting might induce drainage changes and promote or prevent overdeepening.
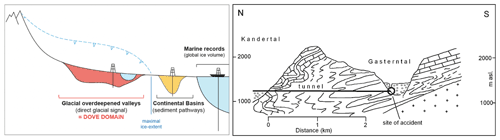
Figure 1Left: schematic sketch (not to scale) of the formation of overdeepened valleys by erosion below glaciers (dashed blue line) reaching deeper than fluvial base level. After glaciation, troughs either are filled with sediment (red) or remain unfilled, thus forming lakes. Together with the distal non-glaciated basins (yellow) and the marine ice-volume records, they provide the sedimentary archives of glaciation history and are the targets of this project. Right: schematic cross-section through Gastern Valley (Bernese Alps, Switzerland) where in 1908 during a railway-tunnel construction, an overdeepened valley was unexpectedly encountered. The breakout of the water-saturated pressurized Quaternary sediments caused 25 casualties (after Preusser et al., 2010).
The sedimentary fillings of overdeepened structures are excellent archives of glaciations and environmental history but have received relatively little scientific attention so far, despite some initiatives on the southern margin of the Scandinavian Ice Sheet (e.g., Gabriel et al., 2003; Jørgensen and Sandersen, 2006; Buechi et al., 2018). In particular, controversy surrounds the age of overdeepening. Buried, deeply incised valleys on the southern side of the Alps likely formed during the Messinian salinity crisis (Bini et al., 1978; Finckh, 1978), but this mechanism actually represents fluvial incision caused by base-level drop. In comparison, beneath the former Scandinavian Ice Sheet, the first overdeepening apparently occurred during the Elsterian glaciation (Kuster and Meyer, 1979). For the Alps, lithostratigraphic records and preliminary dating suggest that some overdeepened valleys were repeatedly occupied and excavated by glaciers (Preusser et al., 2010; Ellwanger et al., 2011; Pomper et al., 2017). Coring and dating first glacial events represent a starting point in the reconstruction of the history of subsequent overdeepening phases. However, only few drill cores have been investigated in detail with regards to the age of original formation (e.g., Dehnert et al., 2012; Fiebig et al., 2014; Schwenk et al., 2022), and the subsequent depositional and erosional history of overdeepened structures in the Alps remains largely unknown.
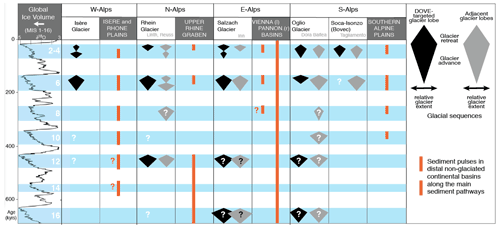
Figure 2Present knowledge of the Quaternary glacial history of the Alps. Correlation of glaciation evidence with marine isotope record (Lisiecki and Raymo, 2005) is tentative; i.e., there is partly little confidence in presence and age as indicated by unfilled time spans and “?”. “Adjacent glacier lobes” indicate glacial catchments not directly targeted by DOVE but in the same Alpine domain that likely responded in a similar manner. Data for W-Alps: Mandier (1988), Nicoud et al. (2002), and Buoncristiani and Campy (2011); N-Alps: Preusser et al. (2011), Ellwanger et al. (2011), and Dehnert et al. (2012); E-Alps: Gabris and Nador (2007), van Husen and Reitner (2011), and Salcher et al. (2012); S-Alps: Bavec et al. (2004), Scardia et al. (2006), Pini et al. (2009), and Gianotti et al. (2015).
The question of the age of overdeepening is causally linked to the controversy of when Alpine glaciers first reached into the foreland and how often (Fig. 2). Penck and Brückner (1901) distinguished four glaciations during the Quaternary, and wiggle matching to deep-sea records predicts the onset of glaciation at ∼ 650 ka (van Husen and Reitner, 2011). For the Rhine Glacier lobe, Ellwanger et al. (2011) suggest the first glaciation reached this area ∼ 1 Ma, but major overdeepening occurred only during three glaciations of the Middle and Late Pleistocene. An age of 870 ka for the onset of glaciation is proposed in northern Italy (Muttoni et al., 2003), with a total of nine glacial advances identified in the Ivrea Morainic Amphitheatre (Gianotti et al., 2015). In Bavaria, gravel deposits of potential glacial origin imply the onset of glaciation during the Early Pleistocene and a minimum of six glaciations, part of which probably comprise more than one glacial advance (Doppler et al., 2011; Fiebig et al., 2011). In northern Switzerland, at least 15 glaciations, starting around 2.5–2.0 Ma ago, are postulated (Preusser et al., 2011). In the Western Alps, information on Early and Middle Pleistocene deposits is scarce. Overdeepening seems to be limited to Alpine valleys and mainly filled with deposits of the last glaciation (Nicoud et al., 2002; Buoncristiani and Campy, 2011); the last maximum of glaciation is expected to date at ∼ 65 ka (e.g., Gribenski et al., 2021). At this time, glaciers were apparently much smaller in Switzerland but still reached the foreland, while in the Austrian Alps, glaciers are expected to have barely reached the main inner-Alpine valleys (cf. Ivy-Ochs et al., 2008).
The apparent differences regarding the timing and extent of glaciations in the Alps are stunning and need to be verified and explained – a main objective of this project. A potential explanation is a different pattern of atmospheric circulation over Europe during glacial times (Florineth and Schlüchter, 2000; Kuhlemann et al., 2008; Monegato et al., 2017). DOVE will provide primary data from ice-proximal sites from overdeepened structures and establish a broad-scale picture by integrating published information from distal, non-glaciated basins in the sphere of the Alps and by comparison with the global marine ice-volume record (Fig. 2). In addition, overdeepened troughs are important for applied aspects such as groundwater-resource management, geothermal exploitation, and radioactive waste disposal. In this regard, geophysical imaging will play an important role in upscaling the information gained in boreholes. In particular, a multi-method approach (Hellman et al., 2017), with modern numerical techniques (Jordi et al., 2018), to integrate borehole data with surface geophysics is desired. Investigation of overdeepened phenomena in the densely populated Alps is hence timely from both a scientific and applied perspective.
On 3–4 April 2013, the ICDP Workshop DOVE “Drilling Overdeepened Alpine Valleys” was held at Villa del Grumello, on the southwestern shore of Lake Como (northern Italy), followed by a field trip on 5 April 2013. The meeting, organized by CNR–IDPA Milan, was attended by 46 scientists from nine European nations (Austria, Denmark, Germany, France, Italy, Slovenia, Sweden, Switzerland, and the Netherlands) and the USA. The outcome of the workshop was a series of hypotheses and scientific questions as well as a drilling strategy for the DOVE drilling program, consisting of a novel approach by investigating several complementary sites along the foreland around an entire mountain range. In the Alps, geophysical surveys and previous boreholes provide a comprehensive picture of the distribution and geometry of overdeepened structures that is not available for any other mountain range. However, available borehole descriptions are not detailed enough for thorough sedimentological interpretation. Furthermore, dating the sedimentary infill has not been possible until very recently, due to the lack of suitable methods. By using a multidisciplinary approach to decipher sedimentary facies and past environmental conditions, and in combination with state-of-the-art dating methods, DOVE will address the following major scientific questions.
-
(Q1) What were the timing and extent of past Alpine glaciations?
The questions of how many glaciations occurred in the Alps and when they started are highly controversial but of fundamental importance to understand the Quaternary dynamics of natural climate and environmental change. Investigating cores will provide major new insights, as they comprise sediments and erosional features that are rarely accessible in outcrops. Furthermore, the timing and extent of past glaciations could vary substantially along and across the Alpine arc. To date, very few projects have applied multidisciplinary approaches, and collaboration between scientists from different regions has been rather limited. Consequently, only an international initiative comprising various case studies on the scale of an entire mountain range will yield sufficient (“non-local”) homogenous information. The key will be to identify cycles of erosion, glacial deposition, and post-glacial infill by carrying out sequence stratigraphy, establishing reliable age control, and relating them to regional and global records (i.e., marine isotope stages, MISs).
-
(Q2) How did atmospheric circulation patterns control ice flow across the Alps?
During glacial periods, the Alps were situated in a particular setting with regards to past atmospheric circulation, and a southward shift of the polar front turned the northern foreland into an Arctic desert, while the southern foreland still received sufficient precipitation to support local forests. This likely caused substantial differences in glacial advances, on both temporal and spatial scales that are still poorly understood (e.g., Luetscher et al., 2015; Monegato et al., 2017). Hinderer et al. (2013) suggest that higher humidity led to stronger glacial conditioning and topographic accentuation of the Western Alps, which is reflected in a modern erosion rate approximately 3-fold higher, with respect to the Eastern Alps. Comparison of the timing and extent of past glaciations through multiple boreholes around the Alps will provide new insights into the regional temperature and precipitation conditions and the related changes in circulation patterns at the temporal scale of several glacial–interglacial periods. The evidence of varying area-specific precipitation patterns collected in this project will then allow for reconstruction of moisture pathways during past glacial periods that will be cross-checked with climate and ice-flow models (e.g., Seguinot et al., 2018).
-
(Q3) How were mountain ranges and their foreland shaped by repetitive glaciations?
There is a gap between established timescales of postglacial sediment transfer and exhumation rates that prohibits elucidating the response of erosional systems to repeated glaciations (Wittman et al., 2007; Salcher et al., 2014, 2021). An expanded circum-Alpine chronology of Quaternary sediments will fill this gap, identify times of high and low erosion rates, and compare them with climate forcing and with the timing of the topographic evolution of the Alps. Repetitive glacial loading and unloading may have caused a flexure of the lithosphere and/or reactivation of basement faults, thus affecting long-term landscape evolution. While numerical modeling provides a useful tool to estimate slip rates along major faults, it needs to be validated by geological records, in order to localize and quantify deformation. The influence of peripheral lithospheric forebulges and ice loading on the reactivation of faults and structures will be evaluated in the context of landscape evolution on glacial–interglacial timescales. Investigating the bedrock contact will help to illuminate the process of overdeepening. Observations so far have distinguished simple or multiple-phase bedrock-erosion surfaces that are characterized sometimes with pieces of dislocated bedrock and/or massive diamicts (LGRB, 2015). DOVE will build upon these results with systematic investigations and by integrating sedimentological and geophysical data.
DOVE will be accompanied by a series of geophysical investigations, before and after the coring, that will be carried out to extend the punctual information provided from boreholes into 2D/3D. In the last years, the sites Tannwald and Basadingen were already covered by high-resolution reflection seismic surveys that imaged a rich seismic stratigraphic architecture of the trough fill and allowed for ideal drill-site location (Burschil et al., 2018, 2019, 2020; Brandt, 2020). Additional geophysical exploration work, comprising complementary methods and investigations at other sites, will be closely linked to borehole information. Thus, DOVE will contribute to a deeper insight into the origin of geophysical signatures. For instance, it can be studied how major unconformities, which are related to the general development of overdeepened structures, are imaged in reflection seismic data (e.g., Büker et al., 1998; Burschil et al., 2018) or how depositional environments are imaged by different seismic facies (e.g., Büker et al., 1998; Reitner et al., 2010). In addition, electrical resistivities can distinguish between different sediment facies (e.g., Rumpel et al., 2009; Reitner et al., 2010). Geophysical surveys will allow for upscaling of the parameters gained in the boreholes to the entire valley extent using structural constraints and geostatistical information for geophysical inversion and contribute towards a better understanding of valley formation and sedimentological processes. This knowledge will also help to improve the interpretation of data from sites where no boreholes are available.
Within DOVE, a series of additional research goals is also foreseen:
-
It is planned to investigate, through pollen analysis, changes in Alpine vegetation structure, plant biodiversity, and phylogeographic patterns, which are likely driven by glacial–interglacial cycles and region-specific climate variability. Within the first DOVE phase, the target is to identify suitable sections in the cores by screening for the biotic content (pollen grains, spores, pollen slide charcoal fragments, algae, and other organic particles).
-
This project also provides the unique opportunity to identify present-day subsurface biological activity (cf. Lee et al., 2010), which will be addressed in more detail, depending on the results of the screening.
-
Once the data acquisition part of the overall DOVE project has reached an advanced stage, separate projects will address modeling landscape response, glacial erosion, and climate.
DOVE uses a multi-method approach by combining geophysics, core sedimentology, laboratory sediment characterization, geochronology, and biological proxy (mainly pollen) that has seen little application in glacial settings so far. To prove the suitability of this concept, a pilot study is described here in some detail that has been executed on cores from Niederweningen (Figs. 3, 4 and 5). This village, located north of Zürich in northern Switzerland, is well known for its rich finds of Pleistocene faunal and floral remains, including several bones of wooly mammoth (Furrer et al., 2007). It is located just 2 km outside the maximum limits of the last glaciation of the foreland (Fig. 6). The gentle valley of Niederweningen is embedded between the easternmost spur of the Jura Mountains to the south (buildup of limestone) and a ridge of Molasse sandstone to the north, which is covered by older Pleistocene glacial deposits. After a first core with a length of 30 m was investigated (Anselmetti et al., 2010), reconstruction of the sedimentary history was executed on a second 93.6 m deep scientific drilling (Dehnert et al., 2012). First, a sedimentary facies model of the subsurface was constructed, based on seismic surveys conducted using an explosive source (Dehnert et al., 2012) and a number of available geotechnical drill holes (Fig. 3). This revealed the presence of a trough carved into Molasse bedrock, reaching almost 200 m below the present surface. As the eastern part of the trough is apparently filled by subglacial deposits and deltaic sediments, the longest sequence with lacustrine deposits was the target of coring using a combination of rotary and of percussion drilling techniques. A large coring diameter of 326 mm was used at the surface part, slimming down to 145 mm at the bottom part of the core. The applied coring techniques allowed for an almost complete recovery and excellent quality of the cores.
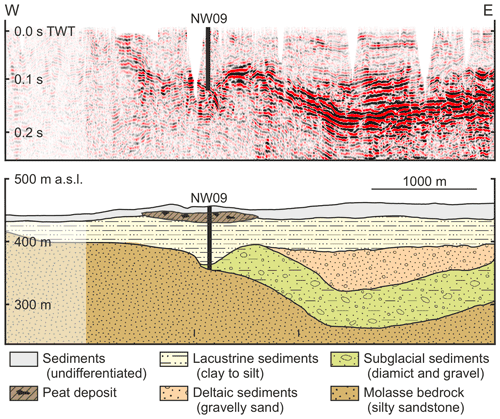
Figure 3Top: seismic survey data along a NW–SE profile parallel to the main valley axis. Bottom: sedimentary facies model of the overdeepened trough of Niederweningen (modified after Dehnert et al., 2012).
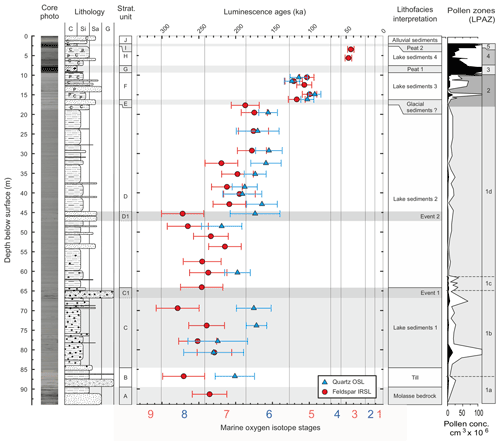
Figure 4Core photograph, grain-size coded stratigraphic column, geochronology, and interpretation of depositional environment of the Niederweningen site (modified after Dehnert et al., 2012) with indicated marine isotope stages at the bottom (Bassinot et al., 1994) and lithofacies interpretation of the stratigraphic units. Pollen concentration data are shown on the right: white areas represent exaggeration × 10 of the pollen concentration curve. LPAZ indicates local pollen assemblage zones: LPAZ 1 – reworked pollen; LPAZ 2 – Alpine meadow, initial phase of Late Glacial reforestation; LPAZ 3 – Eemian or second Early Würmian interstadial; LPAZ 4 – increasingly inundated by water; LPAZ 5: very wet Cyperaceae/moss peat.
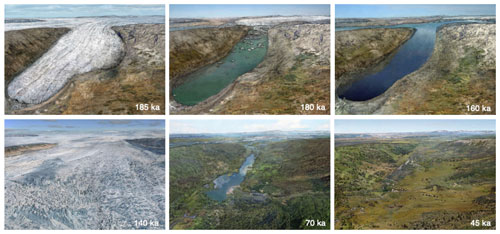
Figure 5Landscape reconstruction with glacial advance and retreat sequences, based on drill-core analysis at Niederweningen (modified after Dehnert et al., 2012).
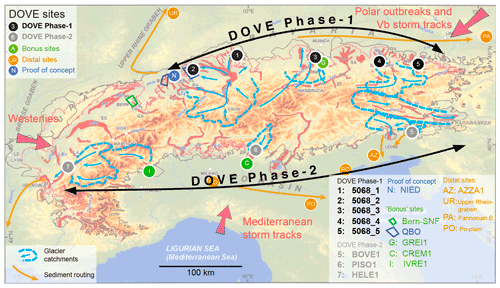
Figure 6Location of primary DOVE Phase 1 sites (black), Nagra QBO sites (11 sites within the blue areas), Phase 2 sites (grey), and bonus sites (green) in the Alpine region, with limit of the Last Glacial Maximum (pink line), maximum limit of Pleistocene glaciation (black line), location of overdeepened structures (red) and pathways of major moisture sources (red arrows) (after Preusser et al., 2010). Indicated are glacial catchments related to the drill-site areas (dashed blue lines) and main paleo-ice-flow directions (blue arrows). Orange circles indicate distal continental basins that lie in the fluvial sediment pathways (arrows) and provide a more continuous but indirect record of glaciations.
After drilling, the liners were cut into 1 m segments and split. The surface of one half-core was carefully prepared, digitally photographed, and described macroscopically to define lithotypes and stratigraphic units. Smear slides (two for each 1 m segment) were prepared to identify the major lithologic constituents, and shear strength was measured every 30 cm using a manual Eijkelkamp vane shear device. The second half-core was used for further subsampling and U-channel extraction. The latter were used to measure bulk magnetic susceptibility and wet bulk density (gamma-ray-based 137Cs source) with a multi-sensor core logger (MSCL). Laboratory analyses included determining total inorganic carbon, total organic carbon, total sulfur, and total nitrogen content as well as laser optical grain-size measurement. The U-channel material was also used to measure the directions and intensities of the natural remanent magnetization. A total of 372 sediment samples were taken, of which 99 were analyzed for their pollen content. From the consolidated (lacustrine) sediments, where the inner parts of the core had not been exposed to light, 29 samples were taken for luminescence dating. The nature of the sediment required application of the fine-grain technique (using silt-size grains 4–11 µm; cf. Preusser et al., 2008). Both optically stimulated luminescence (OSL) of the quartz and infrared stimulated luminescence (IRSL) of the polymineral (feldspar) fraction were used for dating.
The macroscopic description in combination with laboratory sediment analyses allowed for the identification of 10 lithological units within the core (A–J in Fig. 4). This comprises direct glacial (till) and proglacial lacustrine deposits as well as peat and alluvial sediments in the upper part of the sequence. The lithological units were attributed to certain facies based on general appearance and characteristic properties such as the state of consolidation and the presence of drop-stones. Pollen extracted from the sediments has a good to excellent stage of preservation and allows five main local pollen assemblage zones to be defined, including some sub-zones (Fig. 4). The luminescence ages reveal some scatter and offset between OSL and IRSL ages, the latter being mainly higher than the first. This can be explained by the presence of partial bleaching, leading to overestimation of the IRSL age estimates (Dehnert et al., 2012). Hence, the chronology is based on the quartz OSL ages, in combination with information derived from palynology and sediment properties (i.e., unconformities).
The entire dataset gathered during the project was integrated and eventually transferred into visual snapshots of the environmental history, carried out by artists that were advised by members of the scientific team (also available online at http://www.mammutmuseum.ch, last access: 24 September 2022). Figure 5 shows visual snapshots that summarize the glaciation and environmental history of the region, as deduced by the full suite of drill-core analysis. The earliest phase recorded is till resting directly on top of molasse bedrock, which indicates that the glacier either carved out a new basin or at least completely removed all previous Quaternary deposits. Melting of the ice body is reflected by lake sediments, showing the transition from a proximal, drop-stone-dominated facies towards a more distal facies, dominated by silty dropstone-free deposits. According to quartz OSL ages, the area became ice-free around 180 ka. Two breaks in sedimentation are observed within the lake deposits and have major implications for the glaciation history of the area. The first break is identified by disturbed sediment structures, a gradual increase in shear strength, and an offset in both the OSL and IRSL ages. The latter imply this event dates to ∼ 150–140 ka, which is interpreted as the grounding of ice by a cold-based glacier (Dehnert et al., 2012). The second break in sedimentation is indicated by the absence of thermophile pollen that represents the Last Interglacial (Eemian), in concert with a gap of some 10 000 years recorded in the age data (Fig. 4). Luminescence dating and palynology point towards the deposition to be attributed to the second Early Würmian interstadial (Odderade). However, it is not clear whether the gap represents either no deposition or an erosional phase. The upper part of the sequence is composed of peat dated to ∼ 45 ka, hence representing the layer dated in outcrops (Hajdas et al., 2007; Preusser and Degering, 2007) and accompanied with faunal remains (Furrer et al., 2007). The environment is interpreted as wetlands, which represent the final phase of lake development that is finally covered by alluvial deposits transferred downslope during periglacial conditions (Fig. 5). While these artistic reconstructions are based on evidence observed at the site and its surroundings, the visualization includes a certain degree of interpretation.
The DOVE program is designed to be executed in two phases (Fig. 6), with Phase 1 comprising boreholes along the Northern Alpine front and Phase 2 targeting the western and southern margin of the Alps, thus allowing for a complete trans-Alpine dataset. Eventually, a series of drilling and research proposals were funded and integrated with activities investigating the storage of nuclear waste disposals in the Swiss Alpine foreland (National Cooperative for the Disposal of Radioactive Waste) as well as with the geological surveys of Austria, Baden-Württemberg, and Bavaria. Funding granted by ICDP and other partners in 2021 allowed the drilling of two sites (5068_1 (TANN) and 5068_2 (BASA)) and the revisiting of three legacy cores previously drilled at two sites in Bavaria (5068_3 (SCHA) and 5068_4 (FREI) and in an inner-Alpine overdeepening (5068_5 (BADA)). Information from these sites will be complemented by similar drilling programs in glacial overdeepenings in northwestern Switzerland (Fig. 6; QBO – Quaternary drill holes: Gegg et al., 2021; SNF-Bern: Schwenk et al., 2022), and DOVE Phase 1 will eventually comprise more than 20 sites. In addition, ICDP has indicated they will provide funding for the remaining four DOVE sites along the southern transect (DOVE Phase 2), if DOVE Phase 1 is successful and matching funds can be secured.
4.1 ICDP Site 5068_1 (TANN)
Site 5068_1 is situated to the north of Lake Constance, in an area previously covered several times by ice of Rhine Glacier but outside the limits of the last glaciation (Figs. 6, 7). A research drilling in the area carried out in the early 1990s, located ∼ 1300 m south of Site 5068_1 and described in Ellwanger et al. (2011), reached molasse bedrock at a depth of ∼ 209 m. The sequence comprises 13 m of allochthonous molasse underlain by gravel and diamicts of presumed subglacial origin. The ∼ 150 m on top represents bottomsets, which turn into foresets of a lake filling sequence. The middle part of the fine-grained sediments contains pollen reflecting the Holsteinian Interglacial, which implies the formation of the basin is assigned to the Hosskirchian glaciation (Ellwanger et al., 2011).
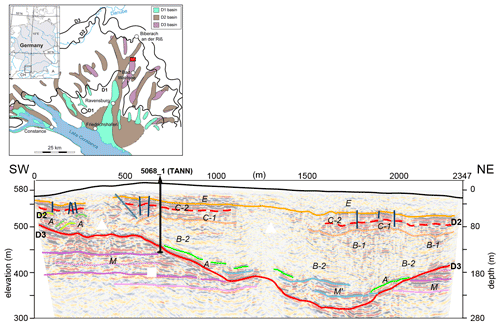
Figure 7Top: maps displaying the location of the DOVE Phase 1 site 5068_1 (TANN) with relation to different generations of overdeepened basins (after Ellwanger et al., 2011). Bottom: interpreted seismic section and superimposed interpretation of profile 1 across the Tannwald basin (from Burschil et al., 2018). Elevation (black line) and faults with offsets > 2 m (dark blue). D3 (base of basin) and D2 are regional discontinuities that separate major sedimentological units. Seismic facies: M upper freshwater molasse, M' allochthonous molasse, A lodgement till, B basin fines, C till sequence, and E fluvial deposits.
Detailed geophysical surveys have contributed to decipher the geometry of the overdeepened structure (Fig. 7; Burschil et al., 2018) and to define the drilling location of Site 5068_1. Drilling began on 6 April 2021 and finished on 3 December 2021. Two flush boreholes (5068_1_A+B) and one core drilling (5068_1_C) were drilled to depths of 163, 155 and 165 m, which reached the base of the Quaternary valley at 153, 154, and 155 m, respectively. The reason for the three boreholes being just 28–40 m apart, which were all subsequently finished as wells with 80 mm PVC casing, is their availability for cross-hole seismic experiments as well as water sampling. The core drilling began using a ramming system, but, especially in the upper 40 m, it was found that either the crown of the corer wore down very quickly or/and a large number of hammer counts were made to achieve a meter of core. Finally, after trying unsuccessfully to ram through highly consolidated sand at 82 m, the coring method was changed to rotary coring. The core drilling took nearly 15 weeks. Drill cores were recovered and stored in opaque PVC liners. During the core drilling, the core catchers were sampled for noble-gas analysis, and geomicrobial samples were taken to study the deep biosphere.
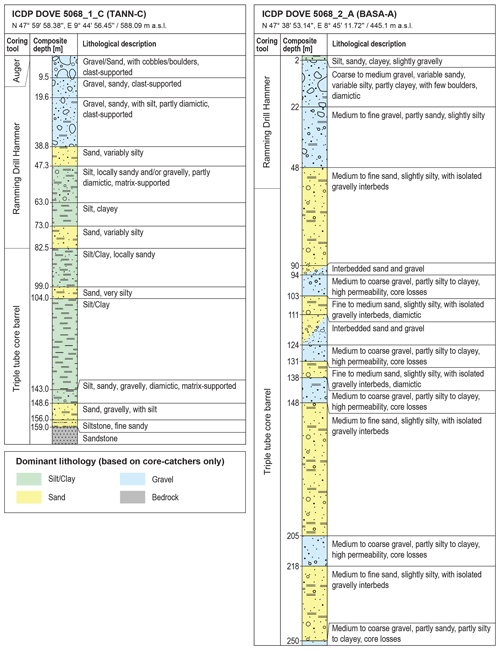
Figure 8Core-catcher-based lithologic logs of ICDP Site 5068_1_C (Tann-C) and 5068_2_A (Basa-A) with indications of drilling technologies.
The core-catcher-based lithologic succession (Fig. 8) shows the upper ∼ 39 m of Hole 5068_1_C consisting of coarse gravelly and partly diamictic sediments interpreted to partly represent direct ice contact from an overriding Rhine Glacier. This coarse succession is underlain by finer grained clayey–silty sediments with occasional sandy layers. These fine-grained lithologies extend downcore to 143 m and are interpreted to have been deposited in a lacustrine setting. From there to the bedrock contact at 159 m, variable lithologies including diamictic and gravelly layers indicate again an ice contact at the base of the overdeepening. Overall, the succession confirms that the trough and its filling represent multiple glacial stages, as supported by the seismic data (Fig. 7).
4.2 ICDP Site 5068_2 (BASA)
Drill site ICDP 5068_2 is located on the Basadingen trough, which is part of the local overdeepened channel system that was formed by the Rhine Glacier during multiple Middle–Late Pleistocene glaciations (Figs. 6, 9). A seismic survey in 2019 (Brandt, 2020) revealed a narrow valley with steep flanks that reaches a maximum depth of up to 300 m (Fig. 9). The seismic sequence analysis indicated a multi-phase stacked sedimentary fill, which was targeted with the selected drill site near an old flush drilling from the 1980s. Drilling operations took place from 25 May to 13 October 2021. The final depth of 253 m was reached using a combined approach of percussion drilling in the upper 60 m and a triple-tube wireline setup below. Bedrock was not reached, but bedrock fragments occurring towards the lowermost meters of the drill core indicate close proximity to the base, as supported by the seismic data. All drill cores were recovered and stored in opaque 1 m long PVC liners. Immediately after recovery, sediment samples were taken from selected cores from the core catchers for geomicrobial and noble-gas porewater analysis. Afterwards, all cores were sealed with caps and labeled and scanned on-site with a MSCL for wet bulk density, magnetic susceptibility, P-wave velocity, and natural gamma radiation. After scanning, the cores were transported to the University of Bern, where they will be stored at 4 ∘C until opening. When drilling operations were completed, a comprehensive wireline logging campaign was carried out in the borehole.
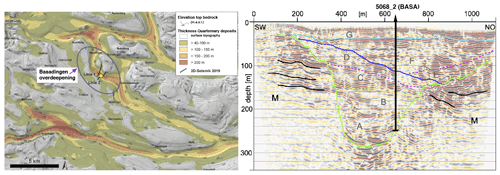
Figure 9Left: location of primary DOVE Phase 1 site 5068_2 (BASA; yellow dot) in the overdeepened trough with a sediment thickness of up to 300 m (bedrock model by Pietsch and Jordan, 2014). The parallel and partly crosscutting overdeepenings likely represent glacial erosional pulses of different ages. They all belong to the western lobe of the Rhine Glacier. Right: seismic Line 2 crossing the Basadingen trough (Brandt, 2020). Note the complex Quaternary sequences (A–G) composing the trough-filling stratigraphy. Bedrock (M) is at ∼ 255 m depth.
A first core-catcher-based lithologic succession (Fig. 8) of Hole 5068_2_A shows a complex stratigraphy with numerous changes between coarse gravelly and partly diamictic sections with sandy lithologies. Only minor amounts of silty lithologies are observed in the core catchers, indicating a rather ice-proximal depositional environment throughout the trough infill. In general, as at Site 5068_1, these initial results and observations support the seismic interpretation, i.e., the occurrence of the proposed multiphase glacial cycles. However, detailed analysis of the cores with a wide range of different proxies, core log, and wireline log data will help to refine the depositional and chronostratigraphic interpretation.
4.3 ICDP Site 5068_3 (SCHA)
This location is situated at the northern edge of the former Isar-Loisach Glacier, just within the limits of the maximum ice extent of the last glaciation. Overdeepening in the region was investigated by Jerz (1987), who identified a basin structure below the present town of Wolfratshausen and adjacent glacial basins in its surroundings. In order to better constrain the detailed shape of these glacial basins, the relative position of the core within the basins and its depositional structures, further geophysical data will be acquired by the Leibniz Institute of Applied Geophysics (LIAG).
Drilling was performed in 2017 by the Bavarian Environment Agency (LfU) and recovered 198.8 m of core. Coring operations had to be stopped for financial reasons, but the base of the Quaternary is assumed to be near the base of the core at ∼ 200 m below the surface. The preliminary sedimentological logging of the core was based on cuttings and investigated the top and bottom parts of the sections. According to these observations, the upper 15 m of the core consist of till, followed by ∼ 100 m of gravel of presumably Rissian and Mindelian age. The lower part of the sequence comprises basin deposits (mainly silt), which is tentatively correlated with either the Mindelian or Günzian glaciation. The cores remained closed until July 2021, when DOVE investigations started. First, all closed cores were scanned at the facilities of the Federal Institute for Geosciences and Natural Resources (BGR) in Berlin Spandau. Subsequently, the cores were cut into two half sections and transported to the sampling repository of the LfU in Hof (Bavaria). Based on the first analysis of core cuttings, the infill of the Schäftlarn Basin spans several glacial cycles. Very recently (late November to early December 2021), the detailed core logging and first sampling of the sequence was started by the DOVE team in the core repository. In 2022, the whole sequence will be sampled and investigated.
4.4 ICDP Site 5068_4 (FREI)
This site is located near the border between Germany and Austria, in an area formerly covered by ice of the Salzach foreland glacier. The research drilling was carried out by the Bavarian Environmental Agency (LfU) in a Quaternary branch basin that was diverted from the main Salzach Glacier basin to the NW. The drilled sequence was first described by Fiebig et al. (2014). The drilling sequence covers 136 m of cores; the Quaternary base was reached at 116 m below the surface. A distinct discontinuity in sedimentation was discovered at ∼ 25 m depth below surface. First age dating was conducted using quartz- and feldspar-based luminescence dating techniques (Fiebig et al., 2014). While these investigations yielded a reliable chronology up to 57 ± 6 ka for the part of the core above the discontinuity, methodological limitations prevented a reliable chronology being established for the part of the core below the discontinuity. Both quartz and feldspar luminescence signals indicated signal saturation, providing only limited age information as all ages beyond 200 ka have to be interpreted as minimum ages. However, methodological progress, especially the development of single-grain luminescence dating techniques using potassium-rich feldspar, may now provide better age control for the older part of the core, with first tests conducted at the Vienna Laboratory for Luminescence dating (VLL) showing promising results. To create a cross-check and an overlap of independent dating methods, additional samples from the lower fine-grained part of the drilling will also be investigated using cosmogenic isotopes.
In addition to the numerical dating approaches, the core will also be reinvestigated in detail with regard to sedimentology, especially including a detailed analysis of the laminated fine lake sediments to further improve the knowledge about the infill and environment of the Pleistocene lake of Freilassing/Neusillersdorf in the Salzach foreland glacier area. Further unconformities in the sequence will be especially addressed by sediment logging and dating in 2022.
4.5 ICDP Site 5068_5 (BADA1)
This site is located in the former Traun Glacier area, inside the limits of the Alps and ∼ 80 km SE of the site 5068_4. The core was first described by van Husen and Mayer (2007) and was subdivided into two sections, with the upper section comprising ∼ 200 m of cover sediments (including glacial diamictons and coarse gravels – interpreted as “Vorstoßschotter” – below) and the lower section consisting of ∼ 700 m of lake sediments from a glacial delta situation. Based on geophysical data (Steinhauser et al., 1985), the Quaternary basin – situated in the midst of the Austrian Alps – is about 1100 m deep and thus reaches a depth below surface comparable to the present-day level of the Dead Sea. Van Husen and Mayer (2007) proposed the formation of this very deep trough below Bad Aussee to be related to dissolution of Alpine salt deposits that are mined near by. A scientific investigation concerning the chronology and mechanism of formation is planned. A new geophysical survey to resolve underground geometry is planned by the Leibniz Institute of Applied Geophysics (LIAG) to investigate the geometry of this dramatically overdeepened structure. The core will be sedimentologically reinvestigated during sample retrieval for age dating starting in early 2022. The start of detailed logging of the cores and sampling for luminescence and cosmogenic dating began in January 2022.
5.1 Geophysical investigations
Data retrieved from drill core or borehole logging data provide important, vertically highly resolved information of the valley fill but only for a limited spatial region. Geophysical measurements are able to project the borehole information (lithology, stratigraphic contacts, and physical properties) into cross-sections and longitudinal sections of overdeepened valley structures. This has already been done by P-wave reflection seismic data for 5068_1 (Burschil et al., 2018) and 5068_2 (Brandt, 2020), providing a very good resolution to image the valley base and some prominent unconformities. For 5068_2, S-wave data (Burschil and Buness, 2020) and two small 3D seismic surveys (P- and S-waves) were also carried out (Buness et al., 2020, 2022; Burschil and Buness, 2020; Burschil et al., 2020, 2022). Aiming to compare the different sites and, thus, catchment areas, 2-D seismic P- and S-wave surveys at the 5068_4 and 5068_5 site are envisaged.
5.1.1 Geophysical downhole logging
For most of the main DOVE and bonus sites (at the legacy sites 5068_3, 5068_3_4, and 5068_3_5, the borehole are not accessible anymore), a geophysical logging program acquired the spectral natural gamma radiation, electrical resistivity, magnetic susceptibility, and acoustic velocity (sonic) logs of the sediments. At the 5068_1 site, further parameters were logged, for example, porosity and density. Depth control and correction of core deformation that results from the process of coring itself (stretching and compression, core loss) will be achieved by comparing data from the multi-sensor core logger with logging data. Therefore, composite lithological profiles of the borehole sites, based on high-resolution core measurements and wireline-logging data, will be used to characterize and interpret the depositional sequences at the individual sites. Advanced geostatistical methods, such as factor and cluster analysis, will support sediment characterization and correlation, especially where complete coring cannot be obtained (Hunze and Wonik, 2009; Hunze et al., 2012; Baumgarten et al., 2014).
The characterization and interpretation of petrophysical properties include sediment composition and textures, cyclicities (if analyzable), and sequence stratigraphy. Gamma ray, magnetic properties, and porosities, etc. are sensitive to changes in clay content and grain size and thus can provide direct textural information; the significance of specific parameters as a proxy for climate or environmental change in these highly dynamic systems needs to be evaluated. The complementing approaches help to identify unconformities and glacial sediment boundaries. The dipmeter, if deployable, provides information about the orientation of sedimentary structures and thus might help to better understand the sedimentation processes. For selected borehole sections, the acoustic borehole televiewer may provide insight into sediment structures. Vertical seismic profiling (VSP) ties borehole information to the acquired surface seismic data. The compaction of the sediments caused by sediment and glacial load will also be analyzed with information from the logs. For instance, trends in compaction might be related to individual glacial cycles. Based on the basic data and derived lithologs, the sediment characteristics of the various drill sites can then be compared with data from existing boreholes and surface geophysics and correlated to identify lateral variations of the sedimentary facies. Finally, the logging data will help to improve the interpretation of surface geophysical data.
5.1.2 Seismic cross-hole tomography
At the 5068_1 site, seismic tomography experiments, funded by the Deutsche Forschungsgemeinschaft, will help to reveal sediment properties and the sedimentation processes during the fill of the basin in more detail. Sedimentation processes may generate an anisotropic sedimentological setting. Different mechanisms that lead to seismic anisotropy of the sediments can be (1) fine layering, (2) clast alignment of fluvial sediments, (3) glacio-tectonic overprint, and (4) over-consolidation by glacial loading. Since seismic near-surface methods lack the resolution of seismic anisotropy to date, we conduct the seismic cross-hole experiment. Site 5068_1 provides a unique opportunity and the ideal setting of three boreholes (∼ 160 m deep) at optimized distances of 28–40 m for such an approach. For the experiment, different source systems that are able to generate P-waves as well as differently polarized S-waves will be deployed. Polarization analyses will give evidence of shear wave splitting and direct access to anisotropy and allow us to identify the arrivals of different wave types. The first arrivals of each wave type are the input for isotropic travel time tomography between the adjacent boreholes. Isotropic full waveform inversion will further increase the resolution and accuracy of seismic velocities. Since the three boreholes enable a comparison of three azimuths of the average P- and S-wave velocities for each sedimentary unit, observed differences in the SV- and SH-wave velocities can give information about seismic anisotropy. Using borehole data, core analysis, and sedimentological interpretation, sedimentological and seismic anisotropy will be integrated to derive sedimentation processes. The findings will be included in a methodical assessment of anisotropic VSP and surface data analysis that can be used at other DOVE locations, where only one borehole is present.
5.1.3 Combined inversion of seismic and resistivity data
Geophysical imaging greatly benefits from a combination of methods that are sensitive to different properties. Electrical resistivity is a parameter that is highly sensitive to groundwater salinity, porosity, and clay content and can thus complement seismic images effectively and efficiently. At 5068_1, an electrical or electromagnetic survey is envisaged for this purpose. The limited resolution inherent in the imaging can be overcome using structural constraints in the inversion (Günther et al., 2011; Doetsch et al., 2012). Furthermore, the ambiguities in the imaging of different parameters can be greatly reduced by coupled cooperative inversion (e.g., Ronczka et al., 2017).
5.2 Core description and sedimentology
Initial analysis at the borehole site and the core repository followed standard ICDP protocols: at the 5068_2 site, a MSCL scanner was used, and at 5068_1 and 5068_2 sites, cores were opened and initially described. Available infrastructure ensures core storage in cold rooms and allows for multi-sensor core logging, cutting, scanning, and sampling, which will be carried out in a consistent manner for all drill cores. A general stratigraphy will be established, and all generated data will be uploaded to the mDIS (ICDP Digital Information System). The data will be integrated with well-log, core-log, and site-survey data, so that all scientists can use them for their respective research topics. After the usual moratorium, data will be available for non-involved scientists, who may submit sample requests to the DOVE operational committee, complemented by the repository curator.
Various sediment properties will be collected to reconstruct the depositional history through lithological, geochemical, physical, and geotechnical investigations. To correlate various sites in different catchment areas, the glacially influenced sediment records will be analyzed, interpreted, and integrated by sequence stratigraphic concepts of a glacial sequence that represents a genetic unit formed during an advance and meltdown cycle (Ellwanger et al., 2011; Powell and Cooper, 2002). These sequences will be recognized by identifying characteristic lithological and geophysical signatures. Combined with the geochronological data, this sequence stratigraphic approach will allow us to correlate sites and sediment provenance, thus addressing the key scientific goals. The proximal sites allow us to identify the direct evidence of glacial advances in the record, i.e., erosional surfaces that mark the transition from erosion to accumulation.
5.2.1 Physical and geotechnical properties
The basic sedimentological description generated during the core-opening sessions by the scientific team will be refined by detailed lithological analysis. Cores will be described using standard sediment classification schemes. Grain-size analysis, combined with observations of sedimentary structures, yields information meltwater volume and flow velocity during deposition. Sedimentological data will be integrated with the data from the multi-sensor core-logger comprising wet bulk density, P-wave velocity, magnetic susceptibility, and natural gamma ray (5068_2 only) in order to define a detailed lithological succession and separate it into various lithotypes. Establishing the glacial sequence stratigraphy in particular requires the recognition of unconformities, which relies on a detailed analysis of potential ice-contact sediments (till layers), including their geotechnical properties. Ice-contact sediments are expected at the base of the overdeepening, where they may be used to reconstruct the mechanisms of bedrock erosion, or within the sedimentary infill, where they can be studied for the mechanisms of basin reactivation. Micromorphological sediment analysis using large thin sections will provide data to evaluate the coupled or decoupled state of the glacier bed that led to the deposition of tills and sorted gravel and sands (Menzies, 2000). This analysis will be matched with X-ray computer tomography of selected half-cores that has proved extremely valuable in analyzing diamictic fabrics and bedrock deformation (Buechi et al., 2017a; Gegg et al., 2020). Glacial sequence boundaries will be identified by investigating the geotechnical properties, such as consolidation state, which is an important parameter to determine whether the sediment was overridden by a glacier. Vane shear testing at regular intervals on the freshly opened surface will provide a quick evaluation of shear strength. Interesting areas may be selected for further geotechnical testing in the laboratory on the basis of the initial measurements. In addition to physico-sedimentological and petrophysical signatures, geochemical and mineralogical patterns provide further criteria to identify major breaks or changes in sedimentation. Total organic and inorganic carbon analysis will be routinely carried out in order to identify potential “warm-climate” lacustrine deposits. Depending on lithology, cores may be analyzed with XRD (X-ray diffraction) for mineralogical patterns and/or XRF (X-ray fluorescence)-scanned for elemental geochemical analysis that may reflect changes in the hydrogeological catchment (Anselmetti et al., 2010; Dehnert et al., 2012). Moreover, provenance studies (heavy mineral analysis and radionuclide compositional analyses) may show changes in the main drainages that could have been triggered by glacial erosion, piracy, or tectonic disturbance.
5.2.2 Screening for biologic proxies
The ultimate aim is to use biogenic remains (e.g., pollen, diatoms) to refine Pleistocene biostratigraphy, the history of mountain life, and the climatic history of the Alps and to trace fluvial erosion and glacial abrasion (reworked biological remains). As previous projects (e.g., Dehnert et al., 2012) have shown, not all fine-grained deposits are suitable archives in this context. In the initial stage we will sample only selected units to test their potential for such analysis. Detailed investigations will be carried out within follow-up proposals, once promising core sections have been identified.
5.3 Sediment dating
A key issue will be to establish reliable and independent chronologies for the different sedimentary records. While biological remains (pollen) may allow us to some extent to determine a stratigraphic setting for the investigated strata, this approach is problematic for the Middle Pleistocene due to the lack of characteristic pollen assemblages. Radiocarbon dating will be applied where appropriate, but most of the scientific questions of this project are very likely beyond its range. Magnetostratigraphy uses the “barcode” pattern of magnetic polarity reversals registered in sediment succession (Opdyke and Channell, 1996). Successful application of magnetostratigraphy to sediments relies on the detection of a number of magnetic polarity reversals or shorter-scale geomagnetic excursions in field intensity, declination, and inclination (secular variations; e.g., Channel, 2006). The main limit of the method is the presence of suitable material, i.e., fine-grained cohesive deposits. To establish a robust chronological framework, magnetostratigraphy will support the application of independent geochronological approaches. The two most promising methods for the present project are luminescence dating and terrestrial cosmogenic nuclides (TCNs). In addition, noble-gas analysis in the porewater might provide information on the age of deposition. We will cross-check results using the three approaches and amend the sampling density as appropriate. Multiple glaciation events and reworking will not substantially reduce the dating accuracy, as recent progress in dating technology allows for the identification of at least the glacial–interglacial period during which sedimentation occurs.
5.3.1 Luminescence dating
This method has already been applied to date the sediment infill of valleys in the foreland of the Alps (e.g., Preusser et al., 2005; Anselmetti et al., 2010; Buechi et al., 2017b). An internal check for reliability is available by comparing ages determined for quartz and feldspar, as the minerals have different physical properties (e.g., Dehnert et al., 2012). Depending on dose rate, standard optically stimulated luminescence (OSL) of quartz and infrared stimulated luminescence (IRSL) of feldspar may be used to date back to about 200 ka. In addition, several luminescence techniques have recently been suggested to be capable of dating older sediments of which the post-IR (pIR)-IRSL method has shown the highest potential to produce reliable ages for the time window back to ∼ 400–500 ka (Buylaert et al., 2012). This approach has also been used in the Upper Rhine Graben, revealing an onset of coarse debris accumulation, presumably related to Alpine glaciations, at at least 450 ka (Preusser et al., 2021).
In general, luminescence ages are associated with uncertainties of ∼ 10 %, and accuracy will be verified by cross-checking different techniques and by comparison with independent approaches; furthermore, interlaboratory comparisons will evidence the reliability of ages. Sampling will be done by using chips of fine-grained material or samples from opaque liners (as planned for all DOVE cores), opened under red-light conditions. Well-established techniques (IRSL/OSL, pIR-IRSL) will be applied using multiple and single-grain approaches and test the performance of different protocols, by carrying out experiments to characterize the luminescence properties (standard tests such as dose recovery, thermal transfer, thermal stability/lifetime, and fading). Based on age estimates from previous dating studies (partly unpublished), samples from the region can be dated back to at least 200 ka (possibly to 500 ka) using both quartz and, in particular, feldspar-based methodological approaches.
5.3.2 Terrestrial cosmogenic nuclides (TCNs)
The determination of burial ages has been used to successfully assign ages to quartz-rich sediments washed into cave systems, for example, in Switzerland by Haeuselmann et al. (2007a, 2015). In addition, glaciofluvial deposits (Deckenschotter) from northern Switzerland were dated to an age range from ∼ 1 to 2 Ma (Claude et al., 2019; Knudsen et al., 2020). In Slovenia, Mihevc et al. (2016) were able to date pebbles from a cave in conglomerate to ∼ 1.8 Ma. In addition, classical sites of Mindelian and Günzian in the Bavarian Alpine foreland have been investigated and found to be between 0.6 and 2.3 Ma years old (Haeuselmann et al., 2007b). Cosmogenic depth-profile ages have been presented for northern Switzerland (Claude et al., 2017), reaching back to 1.5 Ma. The isochron technique (Balco and Rovey, 2008) will be applied to specific samples collected in the project. The actual dating strategy will depend on the sediment succession of each drill core.
5.3.3 Noble gases in porewater
Environmental tracers in the pore fluids of unconsolidated sediments indicate that the porewater that was incorporated during sedimentation can be quantitatively trapped for up to several 100 ka (e.g., Hendry et al., 2005; Hendry and Wassenaar, 2011; Wassenaar and Hendry, 2000). Recently, Tomonaga et al. (2022) demonstrated that fine-grained sediments in overdeepened valleys may preserve porewater that was trapped during sediment deposition; i.e., the sediments and originally deposited (i.e., co-eval) pore fluid were not “separated” and behave as a closed system. Due to their low retention, radiogenic noble gases such as 4He or 40Ar (produced within the solid matrix by the decay of U and Th or 40K, respectively) accumulate in fluids, such as porewater. Therefore, concentrations of radiogenic noble gases (4He, 40Ar) can yield information not only on the residence time of the porewater, but also on the duration of porewater entrapment and thus on the sediment deposition (Brennwald et al., 2013; Tomonaga et al., 2014, 2017). We aim to test this novel technique to porewaters of aquitards to reconstruct the conditions under which the porewater was embedded in these low-permeable sediments.
5.4 Synthesis DOVE Phase 1
Once the drill holes have been analyzed and information on nature and age of infilling and erosional phases are available, data will be compiled and assembled along the northern Alpine transect of Phase 1. Data from the surrounding non-overdeepened landscape, such as tills, wall moraines, or glaciofluvial accumulations, will be integrated into this concept, offering a morphogenetic land-system approach (Ellwanger et al., 2011; LGRB, 2015; Fig. 6). The identified (a)synchronicities will be interpreted in terms of lateral variability of glacier activities along the front of the Alps over the last glacial cycles back into the Middle Pleistocene. These patterns then will be used as constraints for climate and glacier simulations in order to understand the regional dynamics of past atmospheric circulation patterns and their consequences for ice buildup and glacial variations. This new understanding of paleoenvironmental signatures of the past glacial epochs then will serve as a first milestone that will, if successful, justify the planning of DOVE Phase 2.
The ICDP project DOVE will apply a so far infrequently used approach to decipher the origins and environmental context of overdeepened structures in the Alps and their foreland. A key element of the project is investigating a series of drill cores taken from all around the Alps. These will be investigated using unified scientific protocols to allow for direct comparison of the data, which will be stored in online databases. Focus of the first phase of DOVE is the northern foreland of the Alps, where five locations will be addressed in great detail. This comprises the application of classical sedimentological approaches in combination with supporting laboratory analyses (e.g., grain-size determination, micromorphology, X-ray computer tomography). Constraining the age of the sedimentary filling will be done using state-of-the-art geochronological methods, mainly luminescence, TCNs, and noble-gas porewater dating. Suitable sediments will be screened for pollen content that will give insights into environmental conditions but possibly also allow us to establish a biostratigraphy. In addition, two of the recently drilled cores have been sampled to check for potential subsurface biological activity. A key element of DOVE is the application and refinement of geophysical methods that will allow us to identify the subsurface geometry of overdeepened structures. By doing so, this will allow for the upscaling of high-resolution point information gained by analyzing of the sediment sequences of the cores. Once the first phase of DOVE has proven the effectiveness of its approach, it is intended to include cores from the western, southern, and eastern parts of the Alps and by this accomplish a full circum-Alpine approach.
DOVE includes a series of connected objectives. For example, investigating the contact between bedrock and the basal part of the sedimentary filling is expected to add new insights into the mechanisms of formation of overdeepening. Analyzing the facies and age of the sedimentary filling of the structures will allow us to unravel the environmental context. Since several of the overdeepenings remained under-filled for quite some time after their formation, they will also provide information about phases subsequent to the glaciation in which the structure was initially formed. Hence, all this information will help to improve our understanding of the glaciation history of the Alps that, according to recent publications, appears much more complex than often assumed previously. Overall, the data gained during the DOVE project will help to better understand how mountain ranges and their foreland have been shaped by glaciers during the Quaternary. In addition, it has been shown during the past 2 decades that changes in atmospheric circulation very likely had a decisive impact on the glaciation pattern in the Alps. While this is to some extent understood for the Late Pleistocene, knowledge beyond this time is rather limited. Identifying regional differences in the glaciation history during the DOVE project may help to better understand the nature of climate change and its impact during the Quaternary.
All data from this research are published in this paper.
Daniel Ariztegui (Univ. of Geneva, Geneva, Switzerland), Sarah Beraus (LIAG Hanover, Hanover, Germany), Anna-Catharina Brandt (Univ. Hanover, Hanover, Germany), Marius Buechi (Univ. Bern, Bern, Switzerland), Herman Buness (LIAG, Hanover, Germany), Thomas Burschil (BGR Hanover, Hanover, Germany), Andreas Dehnert (ENSI, Brugg, Switzerland), Gaudenz Deplazes (Nagra, Wettingen, Switzerland), Gregor Götzl (GBA, Vienna, Austria), Gustav Firla (BOKU Vienna, Vienna, Austria), Lukas Gegg (Univ. Freiburg, Freiburg, Germany), Hans Rudolf Graf (Dr. von Moos AG, Gächlingen, Switzerland), Katja Heeschen (ICDP-OSG, Potsdam, Germany), Rolf Kipfer (Eawag, Dübendorf, Switzerland), Ernst Kroemer (LfU, Augsburg, Germany), Christopher Lüthgens (BOKU Vienna, Vienna, Austria), Giovanni Monegato (CNR-IGG, Padova, Italy), Stephanie Neuhuber (BOKU Vienna, Vienna, Austria), Roberta Pini (CNR-IGAG, Milan, Italy), Jürgen Reitner (GBA, Vienna, Austria), Bernhard Salcher (Univ. Salzburg, Salzburg, Austria), Sebastian Schaller (Univ. Bern, Bern, Switzerland), Clemens Schmalfuss (BOKU Vienna, Vienna, Austria), Cedric Schmelzbach (ETH Zürich, Zürich, Switzerland), Robert Scholger (Univ. Leoben, Leoben, Austria), Bennet Schuster (Univ. Freiburg, Freiburg, Germany), Andrew Stumpf (Univ. Illinois, Champaign, USA), David C. Tanner (LIAG, Hanover, Germany), Camille Thomas (Univ. Geneva, Geneva, Switzerland), Yama Tomonaga (Eawag, Dübendorf, Switzerland), Ulrike Wieland-Schuster (LGRB, Freiburg, Germany), and Thomas Wonik (LIAG, Hanover, Germany).
All co-authors are co-proponents of the ICDP drilling proposal and contributed to the design and fundraising of the overall DOVE initiative. They all led the discussion on specific topics at the ICDP workshop. Initial text fragments were written by FSA and FP with input from all co-authors and the DOVE science team. The entire text was reviewed by all co-authors.
The contact author has declared that none of the authors has any competing interests.
Publisher’s note: Copernicus Publications remains neutral with regard to jurisdictional claims in published maps and institutional affiliations.
We are grateful for continuing support of the International Scientific Continental Drilling Program (ICDP), including logistic support from the ICDP Operational Support Group. We are grateful to drilling companies, Fretus AG and H. Anger's Söhne GmbH, for providing us with high-quality drill cores in Basadingen and Tannwald, respectively. We also acknowledge strong support by the communities of Basadingen-Schlattingen and Winterstettenstadt, respectively. Technical staff of LIAG assisted with the seismic pre-site surveys and the downhole logging. We acknowledge all ICDP workshop participants in Como, who contributed to realizing this larger DOVE initiative.
This research has been supported by ICDP, the Deutsche Forschungsgemeinschaft (DFG, grant nos. KR2073/3-1, BU 2467/1-2, GA749/5-1, BU2467/3-1, BU 3894/2-1, BU3894/3-1, and PR 957/6-1), Nagra, ENSI, LGRB, LFU, LIAG, BOKU Vienna, and University of Bern.
This paper was edited by Thomas Wiersberg and reviewed by two anonymous referees.
Alley, R., Cuffey, K., and Zoet, L.: Glacial erosion: Status and outlook, Ann. Glaciol., 60, 1–13, https://doi.org/10.1017/aog.2019.38, 2019.
Anselmetti, F. S., Drescher-Schneider, R., Furrer, H., Graf, H. R., Lowick, S. E., Preusser, F., and Riedi, M. A.: A ∼ 180'000 years sedimentation history of a perialpine overdeepened glacial trough (Wehntal, N-Switzerland), Swiss J. Geosci., 103, 345–361, https://doi.org/10.1007/s00015-010-0041-1, 2010.
Balco, G. and Rovey II, C. W.: An isochron method for cosmogenic-nuclide dating of buried soils, American J. Sci., 308, 1083–1114, https://doi.org/10.2475/10.2008.02, 2008.
Bassinot, F. C., Labeyrie, L. D., Vincent, E., Quidelleur, X., Shackleton, N. J., and Lancelot, Y.: The astronomical theory of climate and the age of the Brunhes-Matuyama magnetic reversal, Earth Planet. Sci. Let., 126, 91–108, https://doi.org/10.1016/0012-821X(94)90244-5, 1994.
Bavec, M., Tulaczyk, S. M., Mahan, S. A., and Stock, G. M.: Late Quaternary glaciation of the Upper Soča River Region (Southern Julian Alps, NW Slovenia), Sediment. Geol., 165, 265–283, https://doi.org/10.1016/j.sedgeo.2003.11.011, 2004.
Baumgarten, H., Wonik, T., and Kwiecien, O.: Facies characterization based on physical properties from downhole logging for the sediment record of Lake Van, Turkey, Quaternary Sci. Rev., 104, 85–96, https://doi.org/10.1016/j.quascirev.2014.03.016, 2014.
Bini, A., Cita, M. B., and Gaetani, M.: Southern alpine lakes - hypothesis of an erosional origin related to the Messinian entrenchment, Mar. Geol., 27, 289–302, https://doi.org/10.1016/0025-3227(78)90035-X, 1978.
Brandt, A.-C.: Erkundung des alpinen, glazial-übertieften Basadingen-Beckens mithilfe von P-Wellen-Seismik, BSc thesis, Leibniz Universität Hannover, unpublished, 2020.
Brennwald, M. S., Vogel, N., Scheidegger, Y., Tomonaga, Y., and Kipfer, R.: Noble gases as environmental tracers in porewater of lacustrine or oceanic sediments and in fluid inclusions of stalagmites, in: Advances in Isotope Geochemistry, edited by: Burnard, P., Springer, 123–155, https://doi.org/10.1007/978-3-642-28836-4_6, 2013.
Buechi, M., Frank, S., Graf, H.-R., Menzies, J., and Anselmetti, F. S.: Subglacial emplacement of tills and meltwater deposits at the base of overdeepened bedrock troughs, Sedimentology, 64, 658–685, https://doi.org/10.1111/sed.12319, 2017a.
Buechi, M. W., Lowick, S. E., and Anselmetti, F. S.: Luminescence dating of glaciolacustrine silt in overdeepened basin fills beyond the last interglacial, Quat. Geochronol., 37, 55–67, https://doi.org/10.1016/j.quageo.2016.09.009, 2017b.
Buechi, M. W., Graf, H. R., Haldimann, P., Lowick, S. E., and Anselmetti, F. S.: Multiple Quaternary erosion and infill cycles in overdeepened basins of the northern Alpine foreland, Swiss J. Geosci., 111, 133–167, https://doi.org/10.1007/s00015-017-0289-9, 2018.
Büker, F., Green, A. G., and Horstmeyer, H.: Shallow seismic reflection study of a glaciated valley, Geophysics, 63, 1395–1407, https://doi.org/10.1190/1.1444441, 1998.
Buness, H., Burschil, T., and Tanner, D.: Imaging glacial sediments and tectonics with a small-scale 3-D reflection seismic survey, 26th European Meeting of Environmental and Engineering Geophysics, 7–8 December 2020, online, 1, 1–4, https://doi.org/10.3997/2214-4609.202020094, 2020.
Buness, H., Tanner, D. C., Burschil, T., Gabriel, G., and Wielandt-Schuster, U.: Cuspate-lobate folding in glacial sediments revealed by a small-scale 3-D seismic survey, J. Appl. Geophy., 200, 104614, https://doi.org/10.1016/j.jappgeo.2022.104614, 2022.
Buoncristiani, J.-F. and Campy, M.: Quaternary Glaciations in the French Alps and Jura, Develop. Quat. Sci., 15, 117–126, https://doi.org/10.1016/B978-0-444-53447-7.00010-6, 2011.
Burschil, T. and Buness, H.: S-wave seismic imaging of near-surface sediments using tailored processing strategies, J. Appl. Geophy., 173, 103927, https://doi.org/10.1016/j.jappgeo.2019.103927, 2020.
Burschil, T., Buness, H., Tanner, D., Wielandt-Schuster, U., Ellwanger, D., and Gabriel, G.: High-resolution reflection seismics reveal the structure and the evolution of the Quaternary glacial Tannwald Basin, Near Surf. Geophy., 16, 593–610, https://doi.org/10.1002/nsg.12011, 2018.
Burschil, T., Tanner, D., Reitner, J., Buness, H., and Gabriel, G.: Unravelling the complex stratigraphy of an overdeepened valley with high-resolution reflection seismics: The Lienz Basin (Austria), Swiss J. Geosci., 112, 341–355, https://doi.org/10.1007/s00015-019-00339-0, 2019.
Burschil, T., Buness, H., and Schmelzbach, C.: 3-D Multi-Component S-Wave Survey in the Tannwald Basin: Data processing and component rotation, 26th European Meeting of Environmental and Engineering Geophysics, 7–8 December 2020, online, 1, 1–4, https://doi.org/10.3997/2214-4609.202020103, 2020.
Burschil, T., Buness, H., and Schmelzbach, C.: Near-surface 3-dimensional multi-component source and receiver S-wave survey in the Tannwald Basin, Germany: acquisition and data processing, Near Surf. Geophys., 20, 331–348, https://doi.org/10.1002/nsg.12214, 2022.
Buylaert, J.-P., Jain, M., Murray, A. S., Thomsen, K. J., Thiel, C., and Sohbati, R.: A robust feldspar luminescence dating method for Middle and Late Pleistocene sediments, Boreas, 41, 435–451, https://doi.org/10.1111/j.1502-3885.2012.00248.x, 2012.
Channel, J. E. T: Late Brunhes polarity excursions (Mono Lake, Laschamp, Iceland Basin and Pringle Falls) recorded at ODP Site 919 (Irminger Basin), Earth Planet. Sci. Let., 244, 378–393, https://doi.org/10.1016/j.epsl.2006.01.021, 2006.
Claude, A., Akçar, N., Ivy-Ochs, S., Schlunegger, F., Rentzel, P., Pümpin, C., Tikhomirov, D., Kubik, P. W., Vockenhuber, C., Dehnert, A., Rahn, M., and Schlüchter, C.: Chronology of Quaternary terrace deposits at the locality Hohle Gasse (Pratteln, NW Switzerland), Swiss J. Geosci., 110, 793–809, https://doi.org/10.1007/s00015-017-0278-z, 2017.
Claude, A., Akcar, N., Ivy-Ochs, S., Schlunegger, F., Kubik, P. W., Christl, M., Vockenhuber, C., Kuhlemann, J., Rahn, M., and Schluechter, C.: Changes in landscape evolution patterns in the northern Swiss Alpine Foreland during the mid-Pleistocene revolution, GSA Bulletin, 131, 2056–2078, https://doi.org/10.1130/B31880.1, 2019.
Dehnert, A., Lowick, S. E., Preusser, F., Anselmetti, F. S., Drescher-Schneider, R., Graf, H. R., Heller, F., Horstmeyer, H., Kemna, H. A., Nowaczyk, N. R., Züger, A., and Furrer, H.: Evolution of an overdeepened trough in the northern Alpine Foreland at Niederweningen, Switzerland, Quaternary Sci. Rev., 34, 127–145, https://doi.org/10.1016/j.quascirev.2011.12.015, 2012.
Doetsch, J., Linde, N., Pessognelli, M., Green, A. G., and Günther, T.: Constraining 3-D electrical resistance tomography with GPR reflection data for improved aquifer characterization, J. Appl. Geophys., 78, 68–76, https://doi.org/10.1016/j.jappgeo.2011.04.008, 2012.
Doppler, G., Kroemer, E., Rögner, K., Wallner, J., Jerz, H., and Grottenthaler, W.: Quaternary Stratigraphy of Southern Bavaria, E&G Quaternary Sci. J., 60, 23, https://doi.org/10.3285/eg.60.2-3.08, 2011.
Ellwanger, D., Wielandt-Schuster, U., Franz, M., and Simon, T.: The Quaternary of the southwest German Alpine Foreland (Bodensee-Oberschwaben, Baden-Württemberg, Southwest Germany), E&G Quaternary Sci. J., 60, 22, https://doi.org/10.3285/eg.60.2-3.07, 2011.
Fiebig, M., Ellwanger, D., and Doppler, G.: Pleistocene glaciations of southern Germany, Develop. Quat. Sci., 15, 163–173, https://doi.org/10.1016/B978-0-444-53447-7.00014-3, 2011.
Fiebig, M., Herbst, P., Drescher-Schneider, R., Lüthgens, C., Lomax, J., and Doppler, G.: Some remarks about a new Last Glacial record from the western Salzach foreland glacier basin (Southern Germany), Quatern. Int., 328–329, 107–119, https://doi.org/10.1016/j.quaint.2013.12.048, 2014.
Finckh, P.: Are southern Alpine lakes former Messinian canyons? Geophysical evidence for preglacial erosion in the southern Alpine lakes, Mar. Geol. 27, 289–302, https://doi.org/10.1016/0025-3227(78)90036-1, 1978.
Florineth, D. and Schlüchter, C.: Alpine evidence for atmospheric circulation patterns in Europe during the Last Glacial Maximum, Quaternary Res., 54, 295–308, https://doi.org/10.1006/qres.2000.2169, 2000.
Furrer, H., Graf, H. R., and Mäder, A.: The mammoth site of Niederweningen, Switzerland, Quatern. Int., 164–165, 85–97, https://doi.org/10.1016/j.quaint.2006.10.012, 2007.
Gabriel, G., Kirsch, R., Siemon, B., and Wiederhold, H.: Geophysical investigation of buried Pleistocene subglacial valleys in Northern Germany, J. Appl. Geophys., 53, 159–180, https://doi.org/10.1016/j.jappgeo.2003.08.005, 2003.
Gabris, G. and Nador, A.: Long term fluvial archives in Hungary: response of the Danube and Tisza rivers to tectonic movements and climatic changes during the Quaternary: a review and new synthesis, Quaternary Sci. Rev., 26, 2758–2782, https://doi.org/10.1016/j.quascirev.2007.06.030, 2007.
Gegg, L., Buechi, M. W., Ebert, A., Deplazes, G., Madritsch, H., and Anselmetti, F. S.: Brecciation of glacially overridden palaeokarst (Lower Aare Valley, northern Switzerland): result of subglacial water-pressure peaks?, Boreas 49, 813–827, https://doi.org/10.1111/bor.12457, 2020.
Gegg, L., Deplazes, G., Keller, L., Madritsch, H., Spillmann, T., Anselmetti, F. S., and Buechi, M. W.: 3D morphology of a glacially overdeepened trough controlled by underlying bedrock geology, Geomorphology, 394, 107950, https://doi.org/10.1016/j.geomorph.2021.107950, 2021.
Gianotti, F., Forno, M.G., Ivy-Ochs, S., Monegato, G., Pini, R., and Ravazzi, C.: Stratigraphy of the Ivrea Moranic Amphitheatre (NW Italy): An updated synthesis, Alp. Mediter. Quat., 28, 29–58, 2015.
Gribenski, N., Valla, P., Preusser, F., Roattino, T., Crouzet, C., and Buoncristiani, J.-F.: Out-of-phase Late Pleistocene glacier advances in the western Alps reflect past changes in North Atlantic atmospheric circulation, Geology, 49, 1096–1101, https://doi.org/10.1130/G48688.1, 2021.
Günther, T., Musmann, P., Schaumann, G., and Grinat, M.: Imaging of a fault zone by a large-scale dc resistivity experiment and seismic structural information, 17th EAGE European Meeting of Environmental and Engineering Geophysics, 12–14 September 2011, Leicester, UK, Ext. Abstr., https://doi.org/10.3997/2214-4609.20144450, 2011.
Haeuselmann, P., Granger, D., Jeannin, P.-Y., and Lauritzen, S.-E.: Abrupt glacial valley incision at 0.8 Ma dated from cave deposits in Switzerland, Geology, 35, 143–146, https://doi.org/10.1130/G23094A, 2007a.
Haeuselmann, P., Fiebig, M., Kubik, P. W., and Adrian, H.: A first attempt to date the original “Deckenschotter” of Penck and Brückner with cosmogenic isotopes, Quatern. Int., 164–165, 33–42, https://doi.org/10.1016/j.quaint.2006.12.013, 2007b.
Haeuselmann, P., Mihevc, A., Pruner, P., Horacek, I., Cermak, S., Hercman, H., Sahy, D., Fiebig, M., Hajna, N. Z., and Bosak, P.: Snežna jama (Slovenia): Interdisciplinary dating of cave sediments and implications for landscape evolution, Geomorphology, 247, 10–24, https://doi.org/10.1016/j.geomorph.2014.12.034, 2015.
Hajdas, I., Bonani, G., Furrer, H., Mäder, A., and Schoch, W.: Radiocarbon chronology of the mammoth site at Niederweningen, Switzerland: results from dating bones, teeth, wood, and peat, Quatern. Int., 164–165, 98–105, https://doi.org/10.1016/j.quaint.2006.10.007, 2007.
Hellman, K., Ronczka, M., Günther, T., Wennermark, M., Rücker, C., and Dahlin, T.: Structurally coupled inversion of ERT and refraction seismic data combined with cluster-based model integration, J. Appl. Geophy., 143, 169–181, https://doi.org/10.1016/j.jappgeo.2017.06.008, 2017.
Hendry, M. J. and Wassenaar, L. I.: Millennial-scale diffusive migration of solutes in thick clay-rich aquitards: evidence from multiple environmental tracers, Hydrogeol. J., 19, 259–270, https://doi.org/10.1007/s10040-010-0647-4, 2011.
Hendry, M. J., Kotzer, T. G., and Solomon, D. K.: Sources of radiogenic helium in a clay till aquitard and its use to evaluate the timing of geologic events, Geochim. Cosmochim. Ac., 69, 475–483, https://doi.org/10.1016/j.gca.2004.07.001, 2005.
Hinderer, M., Kastowski, M., Kamelger, A., Bartolini, C., and Schlunegger, F.: River loads and modern denudation of the Alps – A review, Earth Sci. Rev., 118, 11–44, https://doi.org/10.1016/j.earscirev.2013.01.001, 2013.
Hunze, S. and Wonik, T.: Sediment Input into the Heidelberg Basin as determined from Downhole Logs, E&G Quaternary Sci. J., 57, 367–381, https://doi.org/10.3285/eg.57.3-4.5, 2009.
Hunze, S., Gabriel, G., Wiederhold, H., Buness, H., and Wonik, T.: Research borehole Paffrather Mulde 1: characterisation of sediments and structures of the Upper Devonian (Bergisches Land, Germany) by downhole logs, and gravimetric and seismic investigations, Z. dt. Ges. Geowiss., 163, 153–164, https://doi.org/10.1127/1860-1804/2012/0163-0153, 2012.
Huuse, M. and Lykke-Andersen, H.: Overdeepened Quaternary valleys in the eastern Danish North Sea: morphology and origin, Quaternary Sci. Rev., 19, 1233–1253, https://doi.org/10.1016/S0277-3791(99)00103-1, 2000.
Ivy-Ochs, S., Kerschner H., Reuther, A., Preusser, F., Heine, K., Kubik, P. W., Maisch, M., and Schlüchter, C.: Chronology of the last glacial cycle in the European Alps, J. Quat. Sci., 23, 559–573, https://doi.org/10.1002/jqs.1202, 2008.
Jerz, H.: Geologische Karte von Bayern 1 : 25 000, Blatt 8034 Starnberg Süd, Bayerisches Geologisches Landesamt, München, 1987.
Jordi, J., Doetsch, J., Günther, T., Schmelzbach, C., and Robertsson, J. O. A.: Geostatistical regularization operators for geophysical inverse problems on irregular meshes, Geophys. J. Int., 213, 1374–1386, https://doi.org/10.1093/gji/ggy055, 2018.
Jørgensen, F. and Sandersen, P. B. E.: Buried and open tunnel valleys in Denmark – erosion beneath multiple ice sheets, Quaternary Sci. Rev., 25, 1339–1363, https://doi.org/10.1016/j.quascirev.2005.11.006, 2006.
Knudsen, M. F., Nørgaard, J., Grischott, R., Kober, F., Egholm, D. L., Mejer Hansen, T., and Jansen J. D.: New cosmogenic nuclide burial-dating model indicates onset of major glaciations in the Alps during Middle Pleistocene Transition, Earth Planet. Sci. Lett., 549, 116491, https://doi.org/10.1016/j.epsl.2020.116491, 2020.
Kuhlemann, J., Rohling, E. J., Krumrei, I., Kubik, P., Ivy-Ochs, S., and Kucera, M.: Regional synthesis of Mediterranean atmospheric circulation during the Last Glacial Maximum, Science 321, 1338–1340, https://doi.org/10.1126/science.1157638, 2008.
Kuster, H. and Meyer, K.-D.: Glaziäre Rinnen im mittleren und nördlichen Niedersachsen, E&G Quaternary Sci. J., 29, 135–156, https://doi.org/10.3285/eg.29.1.12, 1979.
Lee, H. J., Ho, M. R., Bhuwan, M., Hsu, C. Y., Huang, M. S., Peng, H. L., and Chang, H. Y.: Enhancing ATP-based bacteria and biofilm detection by enzymatic pyrophosphate regeneration, Anal. Biochem., 399, 168–173, https://doi.org/10.1016/j.ab.2009.12.032, 2010.
LGRB (Landesamt für Geologie, Rohstoffe und Bergbau Baden-Württemberg): Lithostratigraphische Entwicklung des baden-württembergischen Rheingletschergebiets: Übertiefte Becken- und Moränen-Landschaft, LGRB-Fachbericht 2015/4, 2015.
Lisiecki, L. E. and Raymo, M. E.: A Pliocene-Pleistocene stack of 57 globally distributed benthic δ18O records, Paleoceanography 20, PA1003, https://doi.org/10.1029/2004PA001071, 2005.
Luetscher, M., Boch, R., Sodemann, H., Spötl, C., Cheng, H., Edwards, R. L., Frisia, S., Hof, F., and Müller, W.: North Atlantic storm track changes during the Last Glacial Maximum recorded by Alpine speleothems, Nat. Commun., 6, 6344, https://doi.org/10.1038/ncomms7344, 2015.
Mandier, P.: Le relief de la moyenne vallée du Rhône au Tertiaire et au Quaternaire, Essai de synthèse paléogéographique. Thèse de Doctorat d'Etat présentée devant l'université de Lyon II., 654 pp., unpublished, 1988.
Menzies, J.: Micromorphological analyses of microfabrics and microstructures indicative of deformation processes in glacial sediments, Geol. Soc. London Spec. Pub., 176, 245–257, https://doi.org/10.1144/GSL.SP.2000.176.01.19, 2000.
Mihevc, A., Bavec, M., Haeuselmann, P., and Fiebig, M.: Dating of the Udin Boršt conglomerate terrace and implications for tectonic uplift in the Northwestern Part of the Ljubljana Basin (Slovenia), Acta Carsologica, 44, 169–176, https://doi.org/10.3986/ac.v44i2.2033, 2016.
Monegato, G., Scardia, G., Hajdas, I., Rizzini, F., and Piccin, A.: The Alpine LGM in the boreal ice-sheets game, Sci. Rep., 7, 2078, https://doi.org/10.1038/s41598-017-02148-7, 2017.
Muttoni, G., Carcano, C., Garzanti, E., Ghielmi, M., Piccin, A., Pini, R., Rogledi, S., and Sciunnach, D.: Onset of major Pleistocene glaciations in the Alps, Geology, 31, 989–992, https://doi.org/10.1130/G19445.1, 2003.
Nicoud, G., Royer, G., Corbin, J.-C., Lemeille, F., and Paillet, A.: Creusement et remplissage de la vallée de l'Isère au Quaternaire récent, Géologie de la France, 4, 39–49, 2002.
Opdyke, N. D. and Channell, J. E. T.: Magnetic Stratigraphy, Academic Press, San Diego, ISBN 0-12-527470-X , 1996.
Penck, A., and Brückner, E.; Die Alpen im Eiszeitalter, Tauchnitz, Leipzig, 1901.
Pietsch, J. and Jordan, P.: Digitales Höhenmodell Basis Quartär der Nordschweiz – Version 2014 und ausgewählte Auswertungen, Technical report, Nagra Arbeitsbericht NAB, 14-02, 2014.
Pini, R., Ravazzi, C., and Donegana, M.: Pollen stratigraphy, vegetation and climate history of the last 215 ka in the Azzano Decimo core (plain of Friuli, north-eastern Italy), Quaternary Sci. Rev., 28, 1268–1290, https://doi.org/10.1016/j.quascirev.2008.12.017, 2009.
Pomper, J., Salcher, B. C., Eichkitz, C., Prasicek, G., Lang, A., Lindner, M., and Götz J.: The glacially overdeepened trough of the Salzach Valley, Austria: Bedrock geometry and sedimentary fill of a major Alpine subglacial basin, Geomorphology, 295, 147–158, https://doi.org/10.1016/j.geomorph.2017.07.009, 2017.
Powell, R. D. and Cooper, J. M.: A glacial sequence stratigraphic model for temperate, glaciated continental shelves, Geol. Soc. London Spec. Pub., 203, 215–244, https://doi.org/10.1144/GSL.SP.2002.203.01.12, 2002.
Preusser, F. and Degering, D.: Luminescence dating of the Niederweningen mammoth site, Switzerland, Quatern. Int., 164–165, 106–112, https://doi.org/10.1016/j.quaint.2006.12.002, 2007.
Preusser, F., Drescher-Schneider, R., Fiebig, M., and Schlüchter, C.: Re-interpretation of the Meikirch pollen record, Swiss Alpine Foreland, and implications for Middle Pleistocene chronostratigraphy, J. Quat. Sci., 20, 607–620, https://doi.org/10.1002/jqs.930, 2005.
Preusser, F., Degering, D., Fuchs, M., Hilgers, A., Kadereit, A., Klasen, N., Krbetschek, M., Richter, D., and Spencer, J. Q. G.: Luminescence dating: basics, methods and applications, E&G Quaternary Sci. J., 57, 95–149, https://doi.org/10.3285/eg.57.1-2.5, 2008.
Preusser, F., Reitner, J., and Schlüchter, C.: Distribution, geometry, age and origin of overdeepened valleys and basins in the Alps and their foreland, Swiss J. Geosci., 103, 407–426, https://doi.org/10.1007/s00015-010-0044-y, 2010.
Preusser, F., Graf, H. R., Keller, O., Krayss, E., and Schlüchter, C.: Quaternary glaciation history of northern Switzerland, E&G Quaternary Sci. J., 60, 21, https://doi.org/10.3285/eg.60.2-3.06, 2011.
Preusser, F., Büschelberger, M., Kemna, H. A., Miocic, J., Mueller, D., and May, J.-H.: Exploring possible links between Quaternary aggradation in the Upper Rhine Graben and the glaciation history of northern Switzerland, Int. J. Earth Sci. (Geol. Rundsch.) 110, 1827–1846, https://doi.org/10.1007/s00531-021-02043-7, 2021.
Reitner, J. M., Gruber, W., Römer, A., and Morawetz, R.: Alpine overdeepenings and paleo-ice flow changes: an integrated geophysical-sedimentological case study from Tyrol (Austria), Swiss J. Geosci., 103, 385–405, https://doi.org/10.1007/s00015-010-0046-9, 2010.
Ronczka, M., Hellman, K., Günther, T., Wisén, R., and Dahlin, T.: Electric resistivity and seismic refraction tomography: a challenging joint underwater survey at Äspö Hard Rock Laboratory, Solid Earth, 8, 671–682, https://doi.org/10.5194/se-8-671-2017, 2017.
Rumpel, H.-M., Binot, F., Gabriel, G., Siemon, B., Steuer, A., and Wiederhold, H.: The benefit of geophysical data for hydrogeological 3D modelling – an example using the Cuxhaven buried valley, Z. dtsch. Ges. Geowiss., 160, 259–269, https://doi.org/10.1127/1860-1804/2009/0160-0259, 2009.
Salcher, B., Meurers, B., Smit, J., Decker, K., Hölzel, M., and Wagreich, M.: Strike-slip tectonics and Quaternary basin formation along the Vienna Basin fault system inferred from Bouguer gravity derivates, Tectonics, 31, TC3004, https://doi.org/10.1029/2011TC002979, 2012.
Salcher, B., Kober, F., Kissling, E., and Willett, S. D.: Glacial impact on short-wavelength topography and long-lasting effects on the denudation of a deglaciated mountain range, Glob. Planet. Change, 115, 59–70, https://doi.org/10.1016/j.gloplacha.2014.01.002, 2014.
Salcher, B., Prasicek, G., Baumann, S., and Kober, F.: Alpine relief limited by glacial occupation time, Geology, 49, 10, 1209–1213, https://doi.org/10.1130/G48639.1, 2021.
Scardia, G., Muttoni, G., and Sciunnach, D.: Subsurface magnetostratigraphy of Pleistocene sediments from the Po Plain (Italy): Constraints on rates of sedimentation and rock uplift, GSA Bulletin, 118, 1299–1312, https://doi.org/10.1130/B25869.1, 2006.
Schwenk, M. A., Schläfli, P., Bandou, D., Gribenski, N., Douillet, G. A., and Schlunegger, F.: From glacial erosion to basin overfill: a 240 m-thick overdeepening–fill sequence in Bern, Switzerland, Sci. Dril., 30, 17–42, https://doi.org/10.5194/sd-30-17-2022, 2022.
Seguinot, J., Ivy-Ochs, S., Jouvet, G., Huss, M., Funk, M., and Preusser, F.: Modelling last glacial cycle ice dynamics in the Alps, The Cryosphere, 12, 3265–3285, https://doi.org/10.5194/tc-12-3265-2018, 2018.
Steinhauser, P., Meurers, B., and Aric, K.: Geophysikalische Detailuntersuchungen der Schwereanomalie von Bad Aussee, Geophysikalischer Forschungsbericht Nr. 18, Institut für Meteorologie und Geophysik, Universität Wien, internal report, 1985.
Tomonaga, Y., Brennwald, M. S., Meydan, A. F., and Kipfer, R.: Noble gases in the sediments of Lake Van – Solute transport and palaeotemperature reconstruction, Quaternary Sci. Rev., 104, 117–126, https://doi.org/10.1016/j.quascirev.2014.09.005, 2014.
Tomonaga, Y., Brennwald, M. S., Livingstone, D. M., Kwiecien, O., Randlett, M.-E., Stockhecke, M., Unwin, K., Anselmetti, F., Beer, J., Haug, G., Schubert, C., Sturm, M., and Kipfer, R.: Porewater salinity reveals past lake-level changes in Lake Van, the Earth's largest soda lake, Sci. Rep., 7, 313, https://doi.org/10.1038/s41598-017-00371-w, 2017.
Tomonaga, Y., Buechi, M., Deplazes, G., and Kipfer, R.: 4He U–Th dating of pore waters from Quaternary sediments of the Swiss Midland, 31st Annual V.M. Goldschmidt Conference, 4–9 July 2021, virtual conference, https://doi.org/10.7185/gold2021.5714, 2021.
Van Husen, D. and Mayer, M.: The hole of Bad Ausssee – An unexpected overdeepened area in NW Steiermark, Austria, Aust. J. Earth Sci., 100, 128–136, 2007.
Van Husen, D. and Reitner, J. M.: An Outline of the Quaternary Stratigraphy of Austria, E&G Quaternary Sci. J., 60, 24, https://doi.org/10.3285/eg.60.2-3.09, 2011.
Wassenaar, L. I. and Hendry, M. J.: Mechanisms controlling the distribution and transport of 14C in a clay-rich till aquitard, Ground Water, 38, 343–349, https://doi.org/10.1111/j.1745-6584.2000.tb00219.x, 2000.
Wittmann, H., Blanckenburg, F. V., Kruesmann, T., Norton, K. P., and Kubik, P.W.: Relation between rock uplift and denudation from cosmogenic nuclides in river sediment in the Central Alps of Switzerland, J. Geophys. Res., 112, F04010, https://doi.org/10.1029/2006JF000729, 2007.