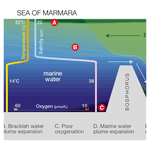
Mediterranean–Black Sea gateway exchange: scientific drilling workshop on the BlackGate project
Wout Krijgsman
Iuliana Vasiliev
Anouk Beniest
Timothy Lyons
Johanna Lofi
Gabor Tari
Caroline P. Slomp
Namik Cagatay
Maria Triantaphyllou
Rachel Flecker
Dan Palcu
Cecilia McHugh
Helge Arz
Pierre Henry
Karen Lloyd
Gunay Cifci
Özgür Sipahioglu
Dimitris Sakellariou
The MagellanPlus workshop “BlackGate” addressed fundamental questions concerning the dynamic evolution of the Mediterranean–Black Sea (MBS) gateway and its palaeoenvironmental consequences. This gateway drives the Miocene–Quaternary circulation patterns in the Black Sea and governs its present status as the world's largest example of marine anoxia. The exchange history of the MBS gateway is poorly constrained because continuous Pliocene–Quaternary deposits are not exposed on land adjacent to the Black Sea or northern Aegean. Gateway exchange is controlled by climatic (glacio-eustatic-driven sea-level fluctuations) and tectonic processes in the catchment as well as tectonic propagation of the North Anatolian Fault Zone (NAFZ) in the gateway area itself. Changes in connectivity trigger dramatic palaeoenvironmental and biotic turnovers in both the Black Sea and Mediterranean domains. Drilling a Messinian to Holocene transect across the MBS gateway will recover high-amplitude records of continent-scale hydrological changes during glacial–interglacial cycles and allow us to reconstruct marine and freshwater fluxes, biological turnover events, deep biospheric processes, subsurface gradients in primary sedimentary properties, patterns and processes controlling anoxia, chemical perturbations and carbon cycling, growth and propagation of the NAFZ, the timing of land bridges for Africa and/or Asia–Europe mammal migration, and the presence or absence of water exchange during the Messinian salinity crisis. During thorough discussions at the workshop, three key sites were selected for potential drilling using a mission-specific platform (MSP): one on the Turkish margin of the Black Sea (Arkhangelsky Ridge, 400 m b.s.f., metres below the seafloor), one on the southern margin of the Sea of Marmara (North İmrali Basin, 750 m b.s.f.), and one in the Aegean (North Aegean Trough, 650 m b.s.f.). All sites target Quaternary oxic–anoxic marl–sapropel cycles. Plans include recovery of Pliocene lacustrine sediments and mixed marine–brackish Miocene sediments from the Black Sea and the Aegean. MSP drilling is required because the JOIDES Resolution cannot pass under the Bosporus bridges. The wider goals are in line with the aims and scope of the International Ocean Discovery Program (IODP) “2050 Science Framework: Exploring Earth by Scientific Ocean Drilling” and relate specifically to the strategic objectives “Earth's climate system”, “Tipping points in Earth's history”, and “Natural hazards impacting society”.
- Article
(18073 KB) - Full-text XML
- BibTeX
- EndNote
Marine gateways are lifelines for restricted basins like the Mediterranean and Black seas, as they govern the regional and global expressions of first-order hydrologic, climatic, and environmental change (Thunell et al., 1988). They play a critical role in the exchange of water, heat, salt, and nutrients between oceans and seas; hence, they impact regional and global climate and marine and terrestrial biodiversity (e.g. Flecker et al., 2015). The complex evolution of the Mediterranean gateways over the past 7 Myr caused environmental challenges that severely impacted marine and terrestrial biota in Eurasia. During the Messinian, the Black Sea gateway opened, and the Atlantic gateway progressively closed as the Mediterranean developed into a largely desiccated saline basin and later into a brackish water “lake-sea” (Lago Mare) during the Messinian salinity crisis (MSC; 5.97–5.33 Ma) (Roveri et al., 2014; Andreetto et al., 2021). Two-way exchange with the Black Sea probably persisted (Vasiliev et al., 2013; Grothe et al., 2020), and its brackish water faunas expanded over the entire Mediterranean as far west as the Malaga Basin adjacent to Gibraltar (Guerra-Merchán et al., 2010). The exact hydrologic fluxes remain uncertain because the dimensions of the Black Sea gateway are poorly constrained (Fig. 1).
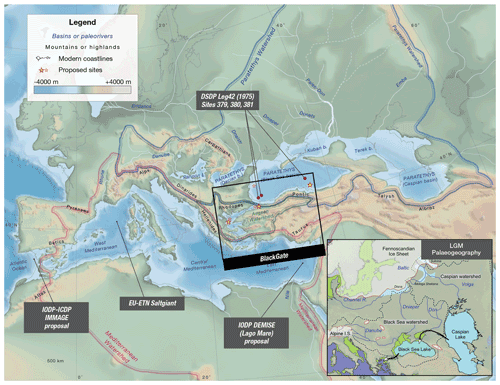
Figure 1Late-Miocene palaeogeography of Eurasia showing the context of BlackGate (after Palcu et al., 2021). The inset shows the palaeogeographic configuration during the Last Glacial Maximum.
The global importance of dynamic Mediterranean connectivity changes is realized by the scientific community, and several projects are currently directed at a better understanding of the Mediterranean gateway system: the European Training Network (ETN) SALTGIANT initiative, funded by the European Union (EU), focuses on the formation of thick evaporite units in the Mediterranean; the International Ocean Discovery Program (IODP)–International Continental Drilling Program (ICDP) amphibious IMMAGE (Investigate Miocene Mediterranean–Atlantic Gateway Exchange) project will be directed at the evolution of the Mediterranean–Atlantic connection; and the IODP project “DEMISE of a salt giant: climatic-environmental transitions during the terminal Messinian Salinity Crisis” aims to unravel halite deformation of the eastern Mediterranean salt deposits. The missing link for a comprehensive understanding of the Mediterranean hydrologic and environmental evolution is the poor comprehension of the hydrological fluxes from the Black Sea domain. For example, the Mediterranean–Black Sea gateway determines the freshwater flux for continental Eurasia (from the Alps in Germany to the Himalayas in western China), which may significantly influence salinity, temperature, and stratification in the Mediterranean. At the same time, these gaps limit our understanding of Black Sea evolution and its broader value in studies of abrupt marine–non-marine transitions and widespread anoxia through history. The gateway influences circulation patterns in the Black Sea (≤2000 m deep) and its status as the world's largest modern example of marine anoxia. This condition dominated the global ocean for the first 90 % of Earth's history as well as intermittently over the last 500 Myr (Lyons et al., 2014), including ocean-scale anoxic events (OAEs) that are often marked by mass extinction.
The Mediterranean region is one of the most vulnerable and rapidly responding areas to present-day climate change. Future warming will likely enhance its salinity and reduce its deep-water formation, all leading to water column stratification and higher mass mortality in marine fauna (Coma et al., 2009). MBS gateway dynamics impact the Mediterranean circulation system as well (Sperling et al., 2003; Filippidi et al., 2016). Salinity variations of Aegean water, largely regulated by lower-salinity-water inflow from the Black Sea, are known to have displaced Mediterranean bottom waters upwards, creating Mediterranean deep-water ventilation and overturn perturbations (Roether et al., 1996; Incarbona et al., 2016). The onset of modern Mediterranean ventilation most likely occurred in the Quaternary, but the influence of Black Sea ingressions on Mediterranean hydrology is still largely unknown. The late-Miocene Mediterranean is characterized by the cyclic development of organic-rich sapropelic layers that reflect climatic and hydrological changes resulting in water column stratification and episodes of anoxic conditions in the deep basins (Rohling et al., 2015). A stratified Mediterranean water mass is also envisaged during the enigmatic Messinian salinity crisis (MSC) halite and gypsum precipitation when the Mediterranean was transformed into a saline basin (García-Veigas et al., 2018). Deciphering the palaeoenvironmental evolution of the Mediterranean–Aegean gateway basin over the past 10 Myr will be crucial to understanding the critical thresholds of ancient and modern corridors, and thus our ability to make environmental and biotic projections for the ocean in the near future under increasing anthropogenic influence.
The Black Sea is the world's largest modern anoxic basin (Arthur and Dean, 1998). This condition results from an estuarine-type circulation driven by riverine inputs and marine Mediterranean inflow through the Bosphorus Strait. The present-day gateway is marked by bottom inflow of dense Aegean water below a surface outflow of fresher Black Sea water. The salty northern Aegean inflow sinks to the deeper parts of the basin and does not mix with the oxygen-rich upper water column. The result is a strongly stratified vertical structure in the Black Sea water column, with 90 % of the deep basin consisting of an anoxic dead zone (Fig. 2; Eckert et al., 2013). A ∼ 100 m thick oxygenated surface layer isolates the strongly sulfidic deep waters from the atmosphere. Bacteria producing hydrogen sulfide (H2S) at high rates make the Black Sea the world's largest H2S (euxinic) body, a condition rare today but common in Earth's past. Recent studies have shown that the thickness of the oxygenated layer in the Black Sea is strongly influenced by freshwater fluxes, increasing temperature, and the ingression of Mediterranean seawater (Capet et al., 2016). Relatively minor changes in connectivity may cause wholesale turnover in basin hydrology, bringing sulfide into the surface waters. Moreover, the geological archives from the Black Sea show multiple episodes of isolation from the Mediterranean during the Messinian to Quaternary (Schrader, 1978; Popov et al., 2006).
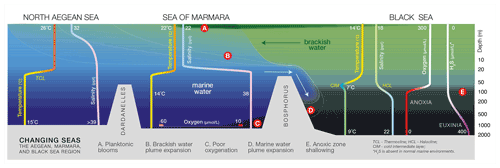
Figure 2Schematic cross section of the water layers in the North Aegean, Sea of Marmara, and Black Sea basins (values after Yakushev et al., 2008; Keskin et al., 2011; Lagaria et al., 2017; and Çağatay et al., 2022). The huge dominant anoxic bottom layer makes the Black Sea the most poisonous sea in the world. Changing the hydrological fluxes may disturb the equilibrium and bring the deadly sulfides towards the surface waters.
Change in climate and associated sea-level variation, in phase with glacial–interglacial cycles, have been suggested as the main drivers of dynamic connectivity, with connections occurring during interglacial sea-level high stands (e.g. Çağatay et al., 2019; Hoyle et al., 2021). The recent sedimentary archives of the Black Sea document its sensitivity to rapid climatic change across a large part of the Eurasian interior (e.g. Fig. 1; Badertscher et al., 2011; Wegwerth et al., 2020). Over the past 7 Myr, however, the Black Sea has experienced a highly dynamic hydrologic history, including episodes during which its catchment tripled in size, incorporating both low-latitude monsoonal systems and high- to mid-latitude glacial cyclicity. The terrestrial signal in sediment cores from this region provides a rare continental record of hemisphere-wide orbital and millennial-scale variability on a scale suitable for robust modelling of the hydrological cycle, a widely acknowledged weakness in general circulation models (GCMs). The long-term records of sea-level change and concomitant consequences for connectivity and coupled biogeochemical processes, like carbon cycling and the development of anoxia, are so far only speculative. In the absence of recent drilling, most of the focus to date is placed on the last glacial–interglacial transition.
In addition, the MBS gateway region is deeply affected by plate tectonic processes of the Africa–Europe collision zone. Subsidence and uplift in the Bosphorus–Marmara–Dardanelles region is strongly driven by North Anatolian Fault activity, a major east–west-running strike-slip fault below the metropolis of Istanbul that shows a record of almost continuous activity throughout the last 7 Myr (Şengör et al., 2005). Tectonically induced palaeoenvironmental changes in the Aegean and Black Sea basins over million-year timescales are not well understood.
The BlackGate workshop was a sequel to the previously funded Magellan workshop BlackSink (2014), which aimed to identify the most suitable drill sites in the Black Sea for exploring the temporal relationships of recurring restriction events, their impact on regional palaeoclimate and palaeoenvironment, and the complex hydro-interactions with the Mediterranean Sea and the global ocean over the last 15 Myr. This prior workshop was an unqualified success, and most attendees (∼40 scientists) concluded that drilling the Miocene to Holocene Black Sea is long overdue and that it would have global importance in our understanding of large-scale anoxia and the causes and consequences of millennial-scale climatic and palaeoenvironmental variability, including implications for the deep biosphere. The workshop was followed by detailed investigations of the old Deep Sea Drilling Project (DSDP) cores (Grothe et al., 2014; Van Baak et al., 2015, 2016; Vasiliev et al., 2015, 2020; Feurdean and Vasiliev, 2019; Golovina et al., 2019; Hoyle et al., 2021) and several field studies of the scattered outcrops in the Black Sea region (Rybkina et al., 2015; Popov et al., 2016; Stoica et al., 2016; Vasiliev et al., 2019), but the key scientific questions about gateway exchange remained. Therefore, we considered it timely to reinvestigate the possibility of drilling the northern Aegean–Marmara–Black Sea transect to comprehensively reconstruct, in a fully interdisciplinary way, the impacts of the east Mediterranean gateway.
The BlackGate workshop was hosted by the Senckenberg Biodiversity and Climate Research Centre in Frankfurt, Germany, and was co-sponsored by the United States Science Advisory Committee (USSAC) for Scientific Ocean Drilling (five scientists from the USA) and SALTGIANT (four early-stage researchers from the EU). It took place from 21 to 24 September 2021. A total of 30 scientists personally attended the workshop (and 7 participated online) from 12 different countries. The participants also represented multiple disciplines, spanning geology, palaeoclimatology, palaeontology, biogeochemistry, tectonics, and reflection seismology, including 11 early-career scientists. The workshop involved numerous scientists with expertise specifically in Black Sea–Mediterranean geology, tectonics, palaeoclimatology, palaeoenvironments, and fundamental biogeochemical processes in the system linked, for example, to changing redox (anoxia), primary production, and salinity. The workshop followed the standard format of concurrent topical breakout sessions on scientific, technical, and funding themes that subsequently reported back to the main group. Breakouts were designed to include complementary disciplines and broadly mixed expertise, but they were also planned with focused expertise in some cases, particularly to address very specific technical questions.
Riding on the success of the BlackGate workshop, we quickly prepared and submitted a preliminary proposal for the IODP 1 October 2021 deadline with the following three themes:
-
generation of high-resolution integrated records of continental-scale climate, sea surface temperature, salinity, anoxia, and thermohaline circulation;
-
impact of Black Sea–Aegean gateway connectivity on biogeochemical processes and sub-seafloor microbial communities; and
-
reconstruction of the detrital provenance and tectonic history of the gateway basins and the surrounding mountains.
To achieve these scientific goals, we propose the use of a mission-specific platform (MSP) to drill three sites: one on the Turkish margin of the Black Sea, one on the southern margin of the Sea of Marmara, and one in the northern Aegean. All sites target Quaternary oxic–anoxic marl–sapropel cycles. Pliocene lacustrine sediments and mixed marine–brackish Miocene sediments will be recovered from the Black Sea and Aegean (Fig. 1).
The gateway is linked to a wide array of critical observations and remaining questions. For example, a recent re-evaluation of the late-Miocene faunal evolution in the northern Aegean basins exposed new palaeogeographic scenarios strikingly different from conventional views, illustrating the need to improve our understanding of the timing and location of critical gateways (Krijgsman et al., 2020). There are no continuous Miocene–Quaternary successions exposed on land in the northern Aegean and Black Sea region. Previous DSDP holes in the Black Sea (Leg 42B) are all disturbed by core breaks and erosional events, and they also include substantial mass transport complexes (Tari et al., 2015), which have only recently been identified and mapped on industrial seismic data. Consequently, there are currently no Miocene–Quaternary cores available for continuous, high-resolution climate reconstruction before the penultimate glacial (Wegwerth et al., 2014). Therefore, the long-term records of hydrology and sea-level change as well as the concomitant consequences for connectivity and coupled biogeochemical processes, carbon cycling, and the development of anoxia are largely speculative. We currently have very little understanding of the impact of major climate events such as the mid-Pliocene warming and the onset of Northern Hemisphere glaciation, locally or regionally. Thus, we are targeting sites on both sides of the MBS gateway because its unique geographic, hydrographic, oceanographic, and tectonic position makes these cores particularly suited to assessing and even quantifying Eurasian palaeoenvironmental processes.
4.1 Theme A: generation of high-resolution integrated records of continental-scale climate, sea surface temperature, salinity, anoxia, and thermohaline circulation
The objectives of Theme A are as follows:
-
to understand the role of global climate and gateway morphology on northern Aegean and Black Sea surface temperature, salinity, anoxia, and thermohaline circulation; and
-
to quantify the time lag between the arrival of Atlantic waters in the Aegean, Marmara, and Black seas at the onset of marine connectivity and evaluate its environmental consequences.
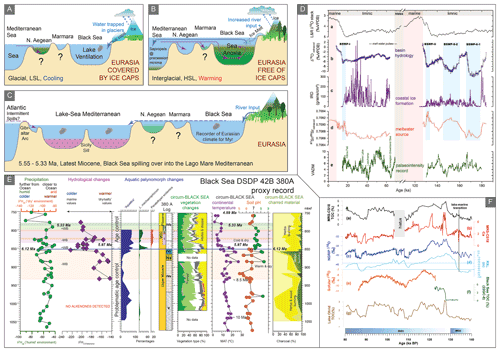
Figure 3Schematic representation of connectivity scenarios in the BlackGate region during (a) glacials and (b) interglacials leading to Black Sea anoxia; (c) Atlantic–Mediterranean–Aegean–Marmara–Black Sea connectivity during the Lago Mare (5.55 to 5.33 Ma) with MSC evaporites shown using pink shading. (d) Example of a study that reflects major changes in the Black Sea hydrology since 185 ka from Major et al. (2006), Nowaczyk et al. (2012, 2021), Kwiecien et al. (2014), and Wegwerth et al. (2014, 2019, 2020). (e) Data from the Black Sea DSDP 42B 380 hole (compiled from Grothe et al., 2014; Vasiliev et al., 2015, 2020; and Feurdean and Vasiliev, 2019). (f) Sea of Marmara records reveal regional and global climate change (Çağatay et al., 2019).
Hydrological exchange between water masses is primarily controlled by salinity and temperature contrasts, but gateway morphology and net evaporation also play roles. During the Miocene–Quaternary, the MBS region experienced repeated, rapid basin-wide salinity fluctuations (e.g. Wegwerth et al., 2014; Çağatay et al., 2019). These reflect the interaction of tectonics, climate, and eustasy as well as changing catchments that at times incorporated both positive and negative water budgets (Gladstone et al., 2007). The process and extent to which these interrelated drivers of gateway exchange interacted remains unquantified. We will explore MBS hydrological interactions by reconstructing the following (Fig. 3):
-
hydrological variations (aquatic vs. terrestrial using biomarker-based compound-specific δ2H);
-
sea surface temperatures (alkenone-based U and isoprenoidal glycerol dialkyl glycerol tetraether (isoGDGT-based) TEX86 proxies. Analysis of clumped isotopes (Δ47), a novel proxy for palaeotemperature reconstructions that, unlike traditional δ18O approaches, is independent of δ18Owater);
-
continental temperatures (primarily soil-derived branched (br) GDGTs), with sea surface temperatures (SSTs) and mean annual air temperatures (MAATs) established in parallel to quantify sea–land temperature contrasts during connectivity changes (e.g. Vasiliev et al., 2013, 2019, 2020);
-
vegetation composition (compound-specific δ13C and δ2H of terrestrial plant-derived long-chain n-alkanes as well as palynology coupled with micro-charcoal; Feurdean and Vasiliev, 2019);
-
anoxia, stratification, and sapropel formation (e.g. isorenieratene; extended archaeol; archaeol; total organic carbon, TOC; ; δ13C; δ15N; and Mn, Fe, Mo, V, Tl, and abundances of other redox-sensitive metals and, in many cases, their isotope relationships; Rohling et al., 2014, 2015);
-
water sources and connectivity proxies ( for continental vs. marine influx; Çağatay et al., 2006; Grothe et al., 2020);
-
environmental change within the water column (δ13C and δ18O on ostracods and, during connection, δ13C and δ18O on foraminifera; Bahr et al., 2005, 2008);
-
of foraminifera to constrain deep-water circulation under different climate and hydrographic forcings (Bialik et al., 2019); and
-
continuous geochemical measurements via core-scanning X-ray fluorescence (XRF) for intervals of interest.
Further, a robust chronology for the MBS system is essential. Although biostratigraphic correlations over the MBS gateway are hampered by the endemic character of Black Sea biota, calcareous nannofossil and dinocyst biostratigraphy coupled with isotope geochemistry can provide ages during marine incursions (Karatsolis et al., 2017; Çağatay et al., 2019; Hoyle et al., 2021). Magnetostratigraphy, radiometric dating, and tephrochronology have all successfully provided age constraints for parts of these sedimentary successions (e.g. Van Baak et al., 2015, 2016; Nowaczyk et al., 2012, 2021; Çağatay et al., 2019; Liu et al., 2020; Wegwerth et al., 2020). We propose the utilization of all of these methods to construct a robust chronological framework for the MBS gateway system over the last 7 Myr.
4.2 Theme B: impact of Black Sea–Aegean gateway connectivity on biogeochemical processes and sub-seafloor microbial communities
The objectives of Theme B are as follows:
-
to understand the linkage between the hydrological connection in the MBS gateway and widespread anoxia, biological turnover, and organic carbon burial in the gateway region;
-
to interrogate environmental variability in the highly sensitive, restricted Black Sea on various timescales ranging from years (varves) to glacial–interglacial (orbital) to millions of years, driven by the interplay of climate, local and global sea level, nutrient inputs, tectonics, macro- and micro-ecology, and the regional water balance; and
-
to elucidate the effects of variations in bottom water oxygen, salinity, and input of organic carbon on microbial communities and biogeochemical processes in a deep-biosphere environment that is strongly influenced by organic-rich sedimentation and pronounced upward fluxes of methane.
The BlackGate drill cores will use state-of-the-art biogeochemical proxies to provide the first reconstruction of water column salinity, redox conditions, temperature, nutrient (re)cycling, primary productivity, (micro)biological communities, and organic matter burial over the entire Miocene–Quaternary interval. While many of these proxies have been developed or refined using samples from the present-day Black Sea often with strong anthropogenic overprints, they have not been applied to the deeper record because of a lack of suitable core samples. Hence, essential aspects of the pre-anthropogenic baseline are missing. Key parameters extracted from sediment records using biogeochemical tools that will complement palaeoenvironmental data collected in Theme A will include the following (Fig. 4):
-
palaeoredox from reactive iron, sulfur, and manganese speciation (Canfield et al., 1996; Poulton and Canfield, 2005; Lenstra et al., 2021); other redox-sensitive trace metals (e.g. molybdenum; Scott and Lyons, 2012; Matthews et al., 2017; Chiu et al., 2022); sulfur isotope systematics (Calvert et al., 1996; Lyons, 1997); carbon / phosphorus ratios () (Algeo and Ingall, 2007); and metal isotopes (Arnold et al., 2004; Owens et al., 2017);
-
palaeosalinity from alkenones (Huang et al., 2021), carbon–sulfur relationships (Lyons and Berner, 1992), and chloride concentrations (Soulet et al., 2010);
-
productivity from organic C and barium where possible (Henkel et al., 2012); and
-
the presence of specific phytoplankton/microbial groups, including those recorded as biomarkers from anoxygenic photosynthetic sulfur oxidizers (Sinninge Damsté et al., 1993) and evidence of anaerobic ammonium oxidation (Kuypers et al., 2003).
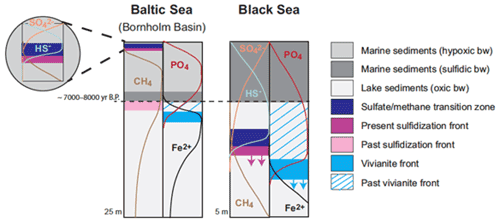
Figure 4Simplified schematic of methane, sulfur, iron, and phosphorus diagenesis in Baltic Sea and Black Sea sediments, illustrating the location of key redox and chemical fronts relevant to post-depositional alteration of both lake and marine sediments (after Dijkstra et al., 2018).
Previous IODP drilling has shown that subsurface seafloor sediments contain a wealth of microorganisms that are metabolically active and drive a range of diagenetic reactions, albeit at low rates (Orcutt et al., 2014). This deep biosphere comprises an important fraction of Earth's total living biomass. We still know relatively little about the metabolism of the microbial groups in the subsurface, including their carbon sources and electron acceptors and donors. These relationships are particularly relevant in gateway regions, where redox, salinity, and other chemical fronts can develop within sediments leading to changes in microbial communities (Marshall et al., 2018), new mineral formation, and diagenetic overprinting of primary depositional signals (Fig. 4; Jørgensen et al., 2001, 2004; Dijkstra et al., 2018). Importantly, although geochemical profiles of solutes and solids can provide key insights into net rates of biogeochemical processes, they do not reveal the specific microbial drivers nor do they reveal cryptic processes in which substrates or products of reactions (e.g. transient intermediates) have short half-lives. For example, methane oxidation in the subsurface may be coupled to reduction of iron oxides, but whether such a reaction truly occurs cannot be proven without insight into the specific microbes involved (Riedinger et al., 2014; Egger et al., 2017).
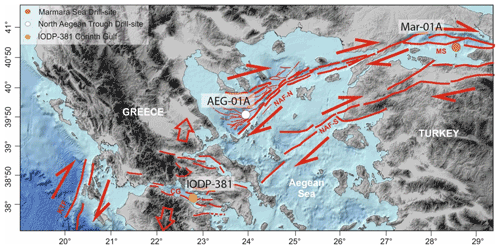
Figure 5The Sea of Marmara–Aegean Sea transform system (map after Sakellariou and Tsampouraki-Kraounaki, 2018), where MS denotes the Sea of Marmara. The red arrows and lines indicate branches of the North Anatolian Fault (NAF) system that are observed on bathymetry and are considered active today.
We will study the impacts of abrupt variations in bottom water oxygen, salinity, and organic carbon input on microbial communities and biogeochemical processes/cycles in the deep subsurface. Detailed geochemical analyses of sediments and porewaters (including C, H, and O isotopes) will reveal the positions of active redox fronts, allowing the separation of primary and secondary signals. Microbiological analyses will include cell counts (Jørgensen et al., 2020), metagenomics (Zinke et al., 2017), measurements of enzyme activities (Schmidt et al., 2021), and the use of various single-cell technologies to link function and genetic identity (Coskun et al., 2018). Our ultimate goal is to determine which microbes are active; how they function; and how they drive geochemical processes in the subsurface, including carbon cycling.
4.3 Theme C: reconstruction of the tectonic evolution of MBS gateway basins and surrounding mountains
The objectives of Theme C are as follows:
-
to unravel the tectonic history of the MBS gateway in Messinian–Quaternary times; and
-
to determine how growth and propagation of strike-slip faults in the continental domain affect gateway dynamics and geohazards.
The MBS gateway is located in a tectonically active region that has been dominated by the westward growth and propagation of the NAFZ over the past 5 Myr (Sengör, 1979; Taymaz et al., 1991; Armijo et al., 1999). Gateway basin geometries and inferred kinematics vary laterally and are heavily affected by NAFZ activity (Fig. 5). The basins in the northern Aegean domain have seen a rapid increase in tectonic activity and opening rate since the late Miocene (Brun et al., 2016), generating increased accommodation space in the past 3–5 Myr (Beniest et al., 2016), coeval with the propagation of the NAFZ into the north Aegean domain (Sakellariou and Tsampouraki-Kraounaki, 2018). Various methods have been used to estimate the age of the gateway sediments in the Aegean domain, including onshore basin stratigraphy (Beniest et al., 2016; Karakitsios et al., 2017), interpolating a Messinian age for large unconformities (Rodriguez et al., 2018; Sakellariou et al., 2018), and using seismic stratigraphy from the deep-sea drilling in the South Aegean Sea (Hsü et al., 1978); however, a robust chronological framework is absent for this region. Recovering sediments that accumulated in the gateway basins will reveal unique information about the evolution of the transform fault system, palaeo-earthquakes associated with their growth (Jolivet and Brun, 2010; Royden and Papanikolaou, 2011), and how they propagate through normal continental and thinned continental crust. Large strike-slip faults like the NAFZ pose a serious threat to society because the gateway region and the geography associated with those faults have been the focus of human activity and urban development for millennia. Specifically, two major population centres, Istanbul and Izmir in north-western Turkey, are located on this fault. A thorough understanding of the gateway's dynamic behaviour with potential temporal patterns is essential for the effective mitigation of seismic hazards.
We will use sediment chronology developed for our cores to ground truth the existing but tentative seismic-stratigraphic framework for the Marmara Trough (Sorlien et al., 2012). These surfaces, which are a sequence of low-stand deltas, have been traced throughout the Sea of Marmara basins and linked to glacial–interglacial cycles. IODP drilling through these strata, in both the Aegean and Marmara domains, coupled with extensive seismic data grids, will provide the chronology necessary for interpretation of transform basin subsidence and fault slip analyses. These results, in turn, will constrain the geometry, kinematics, and segmentation of the main fault branch over the long term, providing a much improved understanding of transform faulting and associated basin evolution. Greater knowledge of the geodynamic context of the MBS gateway basins will eventually shed new light on Miocene plate tectonics, controls on local uplift and subsidence, and active large-scale fault displacements that may lead to slope instability and subsequent mass transfer deposits and earthquake activity.
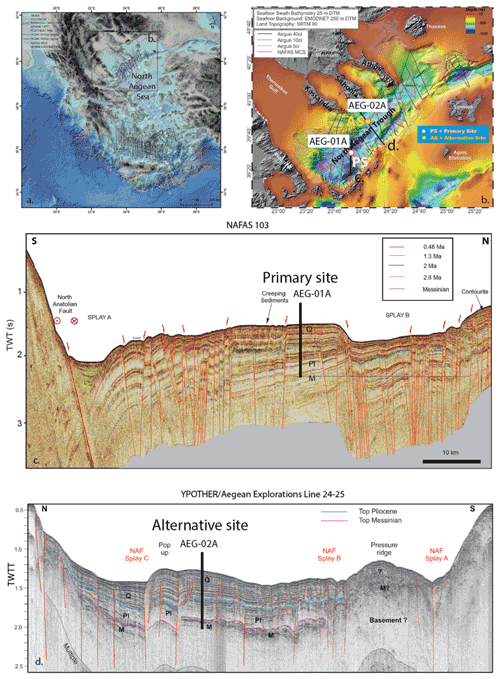
Figure 6Existing seismic data coverage in the North Aegean Sea (the background bathymetry map is sourced from Sakellariou and Tsampouraki-Kraounaki, 2019). High-resolution bathymetry in the northern Aegean Sea with seismic data (Sakellariou et al., 2018). Panel (c) shows seismic line 103 from the North Anatolian Fault in the Aegean Sea (NAFAS) expedition (2017) as well as primary site AEG-01A; the shooting direction was N184∘, the acquisition speed was close to 4.1 knots, the streamer length was 288 m (24 traces, 12.5 m spaced), the offset maximum was 400 m, the shot interval was 12.5 m, the sample rate was 1 ms, the trace length was 5500 ms, and the final bin spacing was 6.25 m. The data were processed with a Kirchhoff post-stack time migration. Seismic units have been identified down to the Messinian (Rodriguez et al., 2018). Panel (d) shows seismic line 13–14, from the YPOTHER/Aegean expeditions (2013–2015) as well as alternative site AEG-02A; the shooting direction was north-north-west–south-south-east, the streamer length was 65 m, the shot interval was 6–6.5 m, and the sample rate was 4000 ms. The data were processed with Kirchhoff post-stack time migration. The proposed ages of the units are given but not verified (Sakellariou et al., 2018). The abbreviations used in the figure are as follows: Q – Quaternary, PI – Pliocene, and M – Messinian.
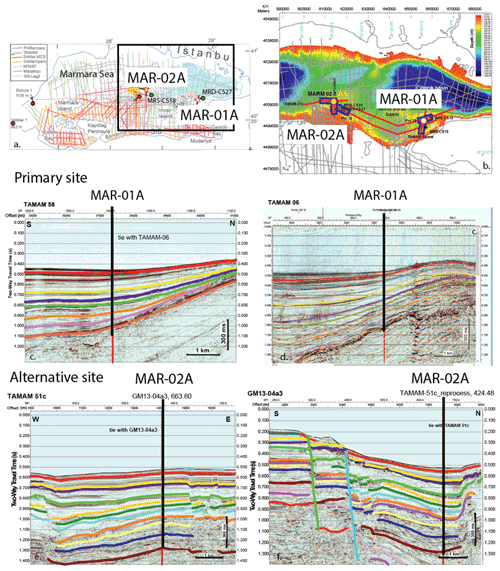
Figure 7(a) MCS data coverage in the Sea of Marmara (after Çağatay et al., 2019): black solid bars show crossing lines for proposed sites MAR-01 and MAR-02, green circles mark holes MRS-CS 18 and MRD-CS 27, and red circles mark deep drill sites by the oil industry. Panel (b) presents a zoomed in map of proposed drill locations. Panels (c) and (d) show seismic line TAMAM 58 and 06 as well as primary site MAR-01A. Panels (e) and (f) show the seismic crossing lines GM13-04a3 and Turkish American Marmara Multichannel (TAMAM) 51 as well as alternative site MAR-02A. All seismic units have been identified but not dated. Shooting of all seismic data in panels (c), (d), (e), and (f) happened during the TAMAM expedition (2008), with a streamer length of 450 m, a shot interval of 12.5 or 18.75 m, and a sample rate of 1 ms. The data were processed with detailed velocity analysis and Kirchhoff post-stack time migration.
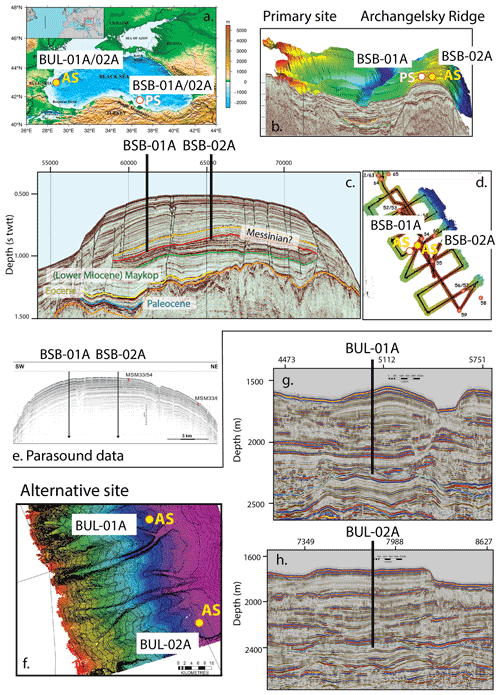
Figure 8(a) Overview map of the Black Sea region with proposed primary and alternative drilling sites (after Avşar and Kutoğlu, 2020). (b) A zoomed in 3D image of proposed drill locations BSB-01A and BSB-2A. (c) Seismic line showing primary sites BSB-01A and BSB-01B; seismic units, characterized by continuous reflectors with various amplitudes, have been identified with expected ages. (d) Overview map of Parasound data acquired in the primary drill location. (e) A high-resolution Parasound profile showing primary sites BSB-01A and BSB-02A. (f) A zoomed in high-resolution bathymetry image showing alternative sites BUL-01A and BUL-02A. (g, h) Seismic line BS-20 2D acquired during “Geology Without Limits” (2011) (Nikishin et al., 2015), showing alternative sites BUL-01A and BUL-02A; the shooting direction was north-west–south-east, the streamer length was 10 200 m, and the sample rate was 4 ms. The data were processed by Kirchhoff pre-stack depth migration.
We have identified three primary and five alternative sites on seismic lines in the northern Aegean (AEG-01A and AEG-02A), the Sea of Marmara (MAR-01A and MAR-02A), and the Black Sea basin (BSB-01A, BSB-02A, BUL-01A, and BUL-02A). Core recovery must be sufficient for high-resolution sampling with minimal gaps. Therefore, we propose the use of an advanced piston corer (APC) to refusal at each site, followed by a rotary core barrel (RCB). Limited core recovery in the single/double sites will be mitigated by the acquisition of downhole logs under open-hole conditions (see below). These data will also be used to generate comprehensive cyclostratigraphic records. More detailed information on the sites used is given in the following:
-
Aegean – primary site is the North Aegean Trough (AEG-01 with ∼650 m penetration; one hole down to 650 m and one hole down to 100 m), yielding a total expected core recovery of 750 m, and the alternative site is AEG-02, which involves a lateral shift in location that shows smaller stratigraphic thickness;
-
Sea of Marmara – preferred site is the North İmrali (MAR-01; one hole down to 400 m), and the alternative site is the MAR-02, which involves a lateral shift in location that shows smaller stratigraphic thickness; and
-
Black Sea – preferred site is the Arkhangelsky Ridge (Turkey) (BSB-01A with ∼400 m penetration with one hole down to 400 m and two holes down to 200 m), yielding a total expected core recovery of 800 m, and the alternative site is the Bulgarian margin (BUL-01A and BUL-02A, ∼750–650 m).
Downhole logging measurements will be important as they can be used to fill gaps in the sedimentary records. Moreover, they offer in situ determinations of the physical properties. At each site, we plan to acquire through-pipe gamma ray logs followed by the following logs collected under open-hole conditions: spectral gamma ray, formation resistivity and magnetic susceptibility, sonic, borehole fluid temperature and conductivity, caliper logs, and vertical seismic profiles (VSPs).
6.1 North Aegean Sea: AEG-01A (primary) and AEG-02A (alternative)
AEG-1A is located on a relative plateau in the southern part of the North Aegean Trough (Fig. 6). At the proposed location, the seismic profile shows a continuous, undisturbed section (AEG-01A; Fig. 6c) at a water depth of 1120 m (see Fig. 6b for details). AEG-2A occupies a plateau in the central deep part of the North Aegean Trough, just south of the Sithonia Peninsula. At the proposed location, the seismic character shows parallel, continuous, undisturbed reflectors (AEG-02A; Fig. 6d) at a water depth of 960 m (see Fig. 6b for details).
6.2 Sea of Marmara: MAR-01A (primary) and MAR-02A (alternative)
MAR-01A is located on a flat plateau in the eastern İmrali Basin near the southern margin of the Sea of Marmara. At the proposed location, the seismic character shows parallel, continuous, undisturbed reflectors (MAR-01A; Fig. 7d) at a water depth of 346 m (see Fig. 7b for details). MAR-02A is located in the western part of İmrali Basin. At the proposed location, the seismic character shows parallel, continuous, undisturbed reflectors (MAR-02A; Fig. 7d) at a water depth of 346 m (see Fig. 7b for details).
6.3 Black Sea: BSB-01A (primary) and BSB-02A (alternative)
The sites are located on the south-eastern end of the north-west–south-east-extending Arkhangelsky Ridge at water depths of 375 and 370 m. The proposed drilling/coring site likely received minimum terrigenous input because it is situated on a relatively high plateau. The existing interpretations include the Messinian unconformity and other significant seismic surfaces in deeper parts of the eastern Black Sea basin (BSB-01A/-02A; Fig. 8c). The geometric relationships between the reflectors and the known geological history of the area were considered when selecting horizons at the proposed drill site.
6.4 BUL-01A and BUL-02A (alternative sites)
The sites are located on the southernmost part of Bulgarian deep-water slope. A large modern 3D seismic data set is available. Few mass transport complexes (MTCs), perhaps at 305–325 m b.s.f., within the Miocene–Quaternary sequence (Tari et al., 2015, 2016; Tishchenko et al., 2021) are expected. Potential drilling location BUL-01A/-02A (Fig. 8g, h) has been selected because of the relatively undeformed sequence between the seafloor and the Messinian unconformity. There is an unconformity at 446 m b.s.f. (Fig. 8h), which may represent up to 20–30 m of missing section within the Pliocene.
No codes or data sets were used in this article.
Konstantina Agiadi (Department of Palaeontology, University of Vienna, Vienna, Austria), Federico Andreetto (Department of Earth Sciences, Utrecht University, Utrecht, the Netherlands), Helge Arz (Marine Geology, Leibniz Institute for Baltic Sea Research Warnemünde, Rostock, Germany), Anouk Beniest (Department of Geosciences, Vrije Universiteit Amsterdam, Amsterdam, the Netherlands), Diksha Bista (NERC Isotope Geosciences Laboratory, British Geological Survey, Keyworth, UK), Francesca Bulian (Department of Geology, University of Salamanca, Salamanca, Spain), Geanina Butiseaca (Senckenberg Biodiversity and Climate Research Centre, Frankfurt am Main, Germany), Namik Cagatay (EMCOL Research Centre, Department of Geological Engineering, Istanbul Technical University, Istanbul, Turkey), Gunay Cifci (Dokuz Eylül Üniversitesi, Deniz Bilimleri ve Teknolojisi Enstitüsü, İnciraltı, İzmir, Turkey), Pinar Ertepinar (Department of Geological Engineering, METU Üniversiteler, Çankaya-Ankara, Turkey), Rachel Flecker (BRIDGE, School of Geographical Sciences and Cabot Institute, University of Bristol, Bristol, UK), Luca Gasperini (Institute of Marine Science, National Research Council ISMAR-CNR, Bologna, Italy), Liviu Giosan (Woods Hole Oceanographic Institution, Woods Hole, USA), Christian Gorini (Sorbonne University, ISTEP, Paris, France), Erhan Gülyüz (Department of Neotectonics and Thermochronology, Institute of Rock Structure and Mechanics of the CAS, Prague, Czech Republic), Pierre Henry (Aix-Marseille University, CNRS, IRD, INRAE, Coll France, CEREGE, Aix-en-Provence, France), Thomas Hoyle (CASP, West Building, Cambridge, UK), Yongsong Huang (Department of Earth, Environmental and Planetary Sciences, Brown University, Providence, USA), Nuretdin Kaymakci (Department of Geological Engineering, METU Üniversiteler, Çankaya-Ankara, Turkey), Wout Krijgsman (Department of Earth Sciences, Utrecht University, Utrecht, the Netherlands), Sergei Lazarev (Carbonate Sedimentology Lab, Department of Geosciences, University of Fribourg, Fribourg, Switzerland), Johanna Lofi (Géosciences Montpellier, CNRS, Université de Montpellier, Montpellier, France), Lucas Lourens (Department of Earth Sciences, Utrecht University, Utrecht, the Netherlands), Timothy Lyons (Department of Earth Sciences, University of California, Riverside, USA), Oleg Mandic (Department of Geology and Paleontology, Natural History Museum Vienna, Vienna, Austria), Cecilia McHugh (Queens College, City University of New York, Flushing, USA), David McInroy (British Geological Survey, Keyworth, UK), Javier Molina-Hernandez (Department of Earth Sciences, Royal Holloway University of London, Egham, UK), Jimmy Moneron (Institute of Earth Sciences, The Hebrew University of Jerusalem, Jerusalem, Israel), Andreas Mulch (Senckenberg Biodiversity and Climate Research Centre, Frankfurt am Main, Germany), Dan Palcu (Instituto Oceanográfico, Universidade do São Paulo, São Paulo, Brazil), Fadl Raad (Géosciences Montpellier, CNRS, Université de Montpellier, Montpellier, France), Dimitris Sakellariou (Institute of Oceanography, Hellenic Centre for Marine Research, Anavyssos, Greece), Özgür Sipahioglu (Exploration Department, Türkiye Petrolleri Anonim Ortakliǧi (TPAO), Çankaya-Ankara, Turkey), Elisabeth Skampa (Faculty of Geology and Geoenvironment, National and Kapodistrian University of Athens, Athens, Greece), Caroline Slomp (Department of Earth Sciences, Utrecht University, Utrecht, the Netherlands), Gabor Tari (OMV Upstream, Exploration, Vienna, Austria), Maria Triantaphyllou (Faculty of Geology and Geoenvironment, National and Kapodistrian University of Athens, Athens, Greece), Iuliana Vasiliev (Senckenberg Biodiversity and Climate Research Centre, Frankfurt am Main, Germany), Antje Wegwerth (Marine Geology, Leibniz Institute for Baltic Sea Research Warnemünde, Rostock, Germany), Frank Wesselingh (Naturalis Biodiversity Center, Leiden, the Netherlands), and Anastasia Yanchilina (Lamont-Doherty Earth Observatory of Columbia University, Palisades, USA).
WK, IV, and AB convened the workshop in Frankfurt. All co-authors contributed to the text and figures that resulted in the submission of a preliminary proposal. DP provided the introductory Figs. 1 and 2. IV, HA, NC, and MT organized Theme A. CPS, TL, and KL wrote Theme B. AB, NC, CM, MT, GC, and PH focused on Theme C. GT, NC, CM, GC, ÖS, and DS provided the seismic lines and location figures. JL wrote the coring strategy. WK, IV, AB, and RF were responsible for revising, editing, and homogenizing the final version of the paper. Participants at the workshop contributed intellectual input.
The contact author has declared that none of the authors has any competing interests.
Publisher's note: Copernicus Publications remains neutral with regard to jurisdictional claims in published maps and institutional affiliations.
The authors wish to thank the Senckenberg Biodiversity and Climate Research Centre in Frankfurt (in particular Andreas Mulch), Germany, for hosting the event and Iryna Yashchenko and Tjitske Vos for their managerial support and assistance. We are also grateful to Lucas Lourens for continued encouragement and constructive suggestions and to Nadine Hallmann and Gilbert Camoin for essential hands-on support with respect to organizing the workshop. Finally, we acknowledge all participants for their constructive input and enthusiasm. Those interested in participating in future BlackGate activities are invited to contact the authors.
This research has been supported by ECORD/IODP (BlackGate2020 grant); the US Science Advisory Committee (five scientists from the USA); and SALTGIANT (four ESRs from the EU), a European project that has received funding from the European Union's Horizon 2020 Research and Innovation programme, within the framework of the Marie Skłodowska-Curie Actions (grant no. 765256). Dan Palcu acknowledges the Fundação de Amparo a Pesquisa do Estado de São Paulo (FAPESP) for financial support (grant no. 2018/20733-6).
This paper was edited by Will Sager and reviewed by Werner Piller and one anonymous referee.
Algeo, T. J. and Ingall, E.: Sedimentary Corg : P ratios, paleocean ventilation, and Phanerozoic atmospheric pO2, Palaeogeogr. Palaeoclimatol. Palaeoecol., 256, 130–155, https://doi.org/10.1016/j.palaeo.2007.02.029, 2007.
Andreetto, F., Aloisi, G., Raad, F., Heida, H., Flecker, R., Agiadi, K., Lofi, J., Blondel, S., Bulian, F., Camerlenghi, A., Caruso, A., Ebner, R., Garcia-Castellanos, D., Gaullier, V., Guibourdenche, L., Gvirtzman, Z., Hoyle, T.M., Meijer, P. T., Moneron, J., Sierro, F. J., Travan, G., Tzevahirtzian, A., Vasiliev, I., and Krijgsman, W.: Freshening of the Mediterranean Salt Giant: controversies and certainties around the terminal (Upper Gypsum and Lago-Mare) phases of the Messinian Salinity Crisis, Earth-Science Rev., 216, 103577, https://doi.org/10.1016/j.earscirev.2021.103577, 2021.
Armijo, R., Meyer, B., Hubert, A., and Barka, A.: Westward propagation of the North Anatolian fault into the northern Aegean: Timing and kinematics, Geology, 27, 267, https://doi.org/10.1130/0091-7613(1999)027<0267:WPOTNA>2.3.CO;2, 1999.
Arnold, G. L., Anbar, A. D., Barling, J., and Lyons, T. W.: Molybdenum Isotope Evidence for Widespread Anoxia in Mid-Proterozoic Oceans, Science, 304, 87–90, https://doi.org/10.1126/science.1091785, 2004.
Arthur, M. A. and Dean, W. E.: Organic-matter production and preservation and evolution of anoxia in the Holocene Black Sea, Paleoceanography, 13, 395–411, https://doi.org/10.1029/98PA01161, 1998.
Avşar, N. B. and Kutoğlu, Ş. H.: Recent sea level change in the Black Sea from satellite altimetry and tide gauge observations, ISPRS Int. J. Geo.-Inf., 9, 185, https://doi.org/10.3390/ijgi9030185, 2020.
Badertscher, S., Fleitmann, D., Cheng, H., Edwards, R. L., Göktürk, O. M., Zumbühl, A., Leuenberger, M., and Tüysüz, O.: Pleistocene water intrusions from the Mediterranean and Caspian seas into the Black Sea, Nat. Geosci., 4, 236–239, https://doi.org/10.1038/ngeo1106, 2011.
Bahr, A., Lamy, F., Arz, H., Kuhlmann, H., and Wefer, G.: Late glacial to Holocene climate and sedimentation history in the NW Black Sea, Mar. Geol., 214, 309–322, 2005.
Bahr, A., Lamy, F., Arz, H. W., Major, C., Kwiecien, O., and Wefer, G.: Abrupt changes of temperature and water chemistry in the late Pleistocene and early Holocene Black Sea, Geochem. Geophy. Geosy., 9, Q01004, https://doi.org/10.1029/2007GC001683, 2008.
Beniest, A., Brun, J. P., Gorini, C., Crombez, V., Deschamps, R., Hamon, Y., and Smit, J.: Interaction between trench retreat and Anatolian escape as recorded by neogene basins in the northern Aegean Sea, Mar. Pet. Geol., 77, 30–42, https://doi.org/10.1016/j.marpetgeo.2016.05.011, 2016.
Bialik, O. M., Frank, M., Betzler, C., Zammit, R., and Waldmann, N. D.: Two-step closure of the Miocene Indian Ocean Gateway to the Mediterranean, Sci. Rep., 9, 1–10, https://doi.org/10.1038/s41598-019-45308-7, 2019.
Brun, J.-P., Faccenna, C., Gueydan, F., Sokoutis, D., Philippon, M., Kydonakis, K., and Gorini, C.: The two-stage Aegean extension, from localized to distributed, a result of slab rollback acceleration, Canadian J. Earth Sci., 53, 1–16, 2016.
Çağatay, M. N., Görür, N., Flecker, R., Sakınç, M., Tünoğlu, C., Ellam, R., Krijgsman, W., Vincent, S., and Dikbaş, A.: Paratethyan–Mediterranean connectivity in the Sea of Marmara region (NW Turkey) during the Messinian, Sediment. Geol., 188–189, 171–187, https://doi.org/10.1016/j.sedgeo.2006.03.004, 2006.
Çağatay, M. N., Eriş, K. K., Makaroğlu, Ö., Yakupoğlu, N., Henry, P., Leroy, S. A. G., Uçarkuş, G., Sakınç, M., Yalamaz, B., Bozyiğit, C., and Kende, J.: The Sea of Marmara during Marine Isotope Stages 5 and 6, Quat. Sci. Rev., 220, 124–141, https://doi.org/10.1016/j.quascirev.2019.07.031, 2019.
Çağatay, M. N., Eris ̧, K. K., and Erdem, Z.: Morphology and Late Pleistocene–Holocene sedimentation of the Strait of Istanbul (Bosphorus): a review, in: Straits and Seaways: Controls, Processes and Implications in Modern and Ancient Systems, edited by: Rossi, V. M., Longhitano, S., Olariu, C., and Chiocci, F., Geological Society, London, Special Publications, 523, https://doi.org/10.1144/SP523-2021-48, 2022.
Calvert, S. E., Thode, H. D., Yeung, D., and Karlin, R. E.: A stable isotope study of pyrite formation in the Late Pleistocene and Holocene sediments of the Black Sea, Geochim. Cosmochim. Ac., 60, 1261–1270, 1996.
Canfield, D. E., Lyons, T. W., and Raiswell, R.: A model for iron deposition to euxinic Black Sea sediments, Am. J. Sci., 296, 818–834. https://doi.org/10.2475/ajs.296.7.818, 1996.
Capet, A., Stanev, E. V., Beckers, J.-M., Murray, J. W., and Grégoire, M.: Decline of the Black Sea oxygen inventory, Biogeosciences, 13, 1287–1297, https://doi.org/10.5194/bg-13-1287-2016, 2016.
Chiu, C. F., Sweere, T. C., Clarkson, M. O., de Souza, G. F., Hennekam, R., and Vance, D.: Co-variation systematics of uranium and molybdenum isotopes reveal pathways for descent into euxinia in Mediterranean sapropels, Earth Planet. Sci Lett., 585, 117527, https://doi.org/10.1016/j.epsl.2022.117527, 2022.
Coma, R., Ribes, M., Serrano, E., Jiménez, E., Salat, J., and Pascual, J.: Global warming-enhanced stratification and mass mortality events in the mediterranean, P. Natl. Acad. Sci. USA, 106, 6176–6181, https://doi.org/10.1073/pnas.0805801106, 2009.
Coskun, Ö. K., Pichler, M., Vargas, S., Gilder, S., and Orsi, W. D.: Linking uncultivated microbial populations and benthic carbon turnover by using quantitative stable isotope probing, Appl. Environ. Microbiol., 84, e01083-18, https://doi.org/10.1128/AEM.01083-18, 2018.
Dijkstra, N., Hagens, M., Egger, M., and Slomp, C. P.: Post-depositional formation of vivianite-type minerals alters sediment phosphorus records, Biogeosciences, 15, 861–883, https://doi.org/10.5194/bg-15-861-2018, 2018.
Eckert, S., Brumsack, H. J., Severmann, S., Schnetger, B., März, C., and Fröllje, H.: Establishment of euxinic conditions in the Holocene Black Sea, Geology, 41, 431–434, https://doi.org/10.1130/G33826.1, 2013.
Egger, M., Hagens, M., Sapart, C. J., Dijkstra, N., van Helmond, N. A. G. M., Mogollón, J. M., Risgaard-Petersen, N., van der Veen, C., Kasten, S., Riedinger, N., Jørgensen, B. B., and Slomp, C. P.: Iron oxide reduction in methane-rich deep Baltic Sea sediments, Geochim. Cosmochim. Ac., 207, 256–276, https://doi.org/10.1016/j.gca.2017.03.019, 2017.
Feurdean, A. and Vasiliev, I.: The contribution of fire to the late Miocene spread of grasslands in eastern Eurasia (Black Sea region), Sci. Rep., 9, 1–7, https://doi.org/10.1038/s41598-019-43094-w, 2019.
Filippidi, A., Triantaphyllou, M. V., and De Lange, G. J.: Eastern-Mediterranean ventilation variability during sapropel S1 formation, evaluated at two sites influenced by deep-water formation from Adriatic and Aegean Seas, Quat. Sci. Rev., 144, 95–106, https://doi.org/10.1016/j.quascirev.2016.05.024, 2016.
Flecker, R., Krijgsman, W., Capella, W., de Castro Martíns, C., Dmitrieva, E., Mayser, J. P., Marzocchi, A., Modestu, S., Ochoa, D., Simon, D., Tulbure, M., van den Berg, B., van der Schee, M., de Lange, G., Ellam, R., Govers, R., Gutjahr, M., Hilgen, F., Kouwenhoven, T., Lofi, J., Meijer, P., Sierro, F. J., Bachiri, N., Barhoun, N., Alami, A. C., Chacon, B., Flores, J. A., Gregory, J., Howard, J., Lunt, D., Ochoa, M., Pancost, R., Vincent, S., and Yousfi, M. Z.: Evolution of the Late Miocene Mediterranean-Atlantic gateways and their impact on regional and global environmental change, Earth-Science Rev., 150, 365–392, https://doi.org/10.1016/j.earscirev.2015.08.007, 2015.
García-Veigas, J., Cendón, D. I., Gibert, L., Lowenstein, T. K., and Artiaga, D.: Geochemical indicators in Western Mediterranean Messinian evaporites: Implications for the salinity crisis, Mar. Geol., 403, 197–214, https://doi.org/10.1016/j.margeo.2018.06.005, 2018.
Gladstone, R., Flecker, R., Valdes, P., Lunt, D., and Markwick, P.: The Mediterranean hydrologic budget from a Late Miocene global climate simulation, Palaeogeogr. Palaeoclimatol. Palaeoecol., 251, 254–267, https://doi.org/10.1016/j.palaeo.2007.03.050, 2007.
Golovina, L. A., Radionova, E. P., Van Baak, C. G. C., Krijgsman, W., and Palcu, D. V.: A Late Maeotian age (6.7–6.3 Ma) for the enigmatic “Pebbly Breccia” unit in DSDP Hole 380A of the Black Sea, Palaeogeogr. Palaeoclimatol. Palaeoecol., 533, 109269, https://doi.org/10.1016/j.palaeo.2019.109269, 2019.
Grothe, A., Sangiorgi, F., Mulders, Y. R., Vasiliev, I., Reichart, G. J., Brinkhuis, H., Stoica, M., and Krijgsman, W.: Black sea desiccation during the Messinian Salinity Crisis: Fact or fiction?, Geology, 42, 563–586, https://doi.org/10.1130/G35503.1, 2014.
Grothe, A., Andreetto, F., Reichart, G.-J., Wolthers, M., Van Baak, C. G. C., Vasiliev, I., Stoica, M., Sangiorgi, F., Middelburg, J. J., Davies, G. R., and Krijgsman, W.: Paratethys pacing of the Messinian Salinity Crisis: Low salinity waters contributing to gypsum precipitation?, Earth Planet. Sci. Lett., 532, 116029, https://doi.org/10.1016/j.epsl.2019.116029, 2020.
Guerra-Merchán, A., Serrano, F., Garcés, M., Gofas, S., Esu, D., Gliozzi, E., and Grossi, F.: Messinian Lago-Mare deposits near the Strait of Gibraltar (Malaga Basin, S Spain), Palaeogeogr. Palaeoclimatol. Palaeoecol., 285, 264–276, https://doi.org/10.1016/j.palaeo.2009.11.019, 2010.
Henkel, S., Mogollón, J. M., Nöthen, K., Franke, C., Bogus, K., Robin, E., Bahr, A., Blumenberg, M., Pape, T., Seifert, R., de Lange, G. J., and Kasten, S.: Diagenetic barium cycling in Black Sea sediments - A case study for anoxic marine environments, Geochim. Cosmochim. Acta, 88, 88–105, https://doi.org/10.1016/j.gca.2012.04.021, 2012.
Hoyle, T. M., Bista, D., Flecker, R., Krijgsman, W., and Sangiorgi, F.: Climate-driven connectivity changes of the Black Sea since 430 ka: Testing a dual palynological and geochemical approach, Palaeogeogr. Palaeoclimatol. Palaeoecol., 561, 110069, https://doi.org/10.1016/j.palaeo.2020.110069, 2021.
Hsü, K. J., Montadert, L., Bernouilli, D., Bizon, G., Cita, M., Erickson, A., Fabricius, F., Garrison, R. E., Kido, R. B., Mellieres, F., Muller, C., and Wright, R. C.: Site 378: Cretan basin, Init. Rep. Deep. Drill. Proj., 42, 321–357, 1978.
Huang, Y., Zheng, Y., Heng, P., Giosan, L., and Coolen, M. J. L.: Black Sea paleosalinity evolution since the last deglaciation reconstructed from alkenone-inferred Isochrysidales diversity, Earth Planet. Sci. Lett., 564, 116881, https://doi.org/10.1016/j.epsl.2021.116881, 2021.
Incarbona, A., Martrat, B., Mortyn, P. G., Sprovieri, M., Ziveri, P., Gogou, A., Jordà, G., Xoplaki, E., Luterbacher, J., Langone, L., Marino, G., Rodríguez-Sanz, L., Triantaphyllou, M., Di Stefano, E., Grimalt, J. O., Tranchida, G., Sprovieri, R., and Mazzola, S.: Mediterranean circulation perturbations over the last five centuries: Relevance to past Eastern Mediterranean Transient-type events, Sci. Rep., 6, 1–10, https://doi.org/10.1038/srep29623, 2016.
Jolivet, L. and Brun, J.-P.: Cenozoic geodynamic evolution of the Aegean, Int. J. Earth Sci., 99, 109–138, https://doi.org/10.1007/s00531-008-0366-4, 2010.
Jørgensen, B. B., Weber, A., and Zopfi, J.: Sulfate reduction and anaerobic methane oxidation in Black Sea sediments, Deep Sea Res. Part I Oceanogr. Res. Pap., 48, 2097–2120, 2001.
Jørgensen, B. B., Böttcher, M. E., Lüschen, H., Neretin, L. N., and Volkov, I. I.: Anaerobic methane oxidation and a deep H2S sink generate isotopically heavy sulfides in Black Sea sediments, Geochim. Cosmochim. Ac., 9, 2095–2118, 2004.
Jørgensen, B. B., Andrén, T., and Marshall, I. P. G.: Sub-seafloor biogeochemical processes and microbial life in the Baltic Sea, Environ. Microbiol., 22, 1688–1706, https://doi.org/10.1111/1462-2920.14920, 2020.
Karakitsios, V., Cornee, J., Tsourou, T., Moissette, P., Kontakiotis, G., Agiadi, K., Manoutsoglou, E., Triantaphyllou, M., and Koskeridou, E.: Messinian salinity crisis record under strong freshwater input in marginal, intermediate, and deep environments: the case of the North Aegean. Paleogr. Paleoclimatol. Paleoecol. 485, 316–335, https://doi.org/10.1016/j.palaeo.2017.06.023, 2017.
Karatsolis, B. T., Triantaphyllou, M. V., Dimiza, M. D., Malinverno, E., Lagaria, A., Mara, P., Archontikis, O., and Psarra, S.: Coccolithophore assemblage response to Black Sea Water inflow into the North Aegean Sea (NE Mediterranean), Cont. Shelf Res., 149, 138–150, https://doi.org/10.1016/j.csr.2016.12.005, 2017.
Keskin, C., Ordines, F., Guijarro, B., and Massutí, E.: Comparison of fish assemblages between the Sea of Marmara and the Aegean Sea (north-eastern Mediterranean), J. Mar. Biol. Assoc. United Kingdom, 91, 1307–1318, https://doi.org/10.1017/S0025315410002213, 2011.
Krijgsman, W., Palcu, D. V., Andreetto, F., Stoica, M., and Mandic, O.: Changing seas in the late Miocene Northern Aegean: A Paratethyan perspective to Mediterranean stratigraphy, Earth Sci. Rev., 210, 103386, https://doi.org/10.1016/j.earscirev.2020.103386, 2020.
Kuypers, M. M. M., Sliekers, A. O., Lavik, G., Schmid, M., Jørgensen, B. B., Kuenen, J. G., Sinninge Damsté, J. S., Strous, M., and Jetten, M. S. M.: Anaerobic ammonium oxidation by anammox bacteria in the Black Sea, Nature, 422, 608–611, 2003.
Kwiecien, O., Stockhecke, M., Pickarski, N., Heumann, G., Litt, T., Sturm, M., Anselmetti, F., Kipfer, R., and Haug, G. H.: Dynamics of the last four glacial terminations recorded in Lake Van, Turkey, Quat. Sci. Rev., 104, 42–52, https://doi.org/10.1016/j.quascirev.2014.07.001, 2014.
Lagaria, A., Mandalakis, M., Mara, P., Frangoulis, C., Karatsolis, B. T., Pitta, P., Triantaphyllou, M., Tsiola, A., and Psarra, S.: Phytoplankton variability and community structure in relation to hydrographic features in the NE Aegean frontal area (NE Mediterranean Sea), Cont. Shelf Res., 149, 124–137, https://doi.org/10.1016/j.csr.2016.07.014, 2017.
Lenstra, W. K., Klomp, R., Molema, F., Behrends, T., and Slomp, C. P.: A sequential extraction procedure for particulate manganese and its application to coastal marine sediments, Chem. Geol., https://doi.org/10.1016/j.chemgeo.2021.120538, 2021.
Liu, J., Nowaczyk, N. R., Panovska, S., Korte, M., and Arz, H. W.: The Norwegian-Greenland Sea, the Laschamps, and the Mono Lake Excursions Recorded in a Black Sea Sedimentary Sequence Spanning From 68.9 to 14.5 ka, J. Geophys. Res.-Sol. Ea., 125, e2019JB019225, https://doi.org/10.1029/2019JB019225, 2020.
Lyons, T. W.: Sulfur isotopic trends and pathways of iron sulfide formation in upper holocene sediments of the anoxic black sea, Geochim. Cosmochim. Ac., 61, 3367–3382, https://doi.org/10.1016/S0016-7037(97)00174-9, 1997.
Lyons, T. W. and Berner, R. A.: Carbon-sulfur-iron systematics of the uppermost deep-water sediments of the Black Sea, Chem. Geol., 99, 1–27, 1992.
Lyons, T. W., Reinhard, C. T., and Planavsky, N. J.: The rise of oxygen in Earth's early ocean and atmosphere, Nature, 506, 307–315, https://doi.org/10.1038/nature13068, 2014.
Major, C. O., Goldstein, S. L., Ryan, W. B. F., Lericolais, G., Piotrowski, A. M., and Hajdas, I.: The co-evolution of Black Sea level and composition through the last deglaciation and its paleoclimatic significance, Quat. Sci. Rev., 25, 2031–2047, https://doi.org/10.1016/j.quascirev.2006.01.032, 2006.
Marshall, I. P. G., Karst, S. M., Nielsen, P. H., and Jørgensen, B. B.: Metagenomes from deep Baltic Sea sediments reveal how past and present environmental conditions determine microbial community composition, Mar. Genomics, 37, 58–68, https://doi.org/10.1016/j.margen.2017.08.004, 2018.
Matthews, A., Azrieli-Tal, I., Benkovitz, A., Bar-Matthews, M., Vance, D., Poulton, S. W., Teutsch, N., Almogi-Labin, A., and Archer, C.: Anoxic development of sapropel S1 in the Nile Fan inferred from redox sensitive proxies, Fe speciation, Fe and Mo isotopes, Chem. Geol., 475, 24–39, https://doi.org/10.1016/j.chemgeo.2017.10.028, 2017.
Nikishin, A. M., Okay, A. I., Tüysüz, O., Demirer, A., Amelin, N., and Petrov, E.: The Black Sea basins structure and history: new model based on new deep penetration regional seismic data. Part 1: Basins structure and fill, Mar. Pet. Geol., 59, 638–655, 2015.
Nowaczyk, N. R., Arz, H. W., Frank, U., Kind, J., and Plessen, B.: Dynamics of the Laschamp geomagnetic excursion from Black Sea sediments, Earth Planet. Sci. Lett., 351–352, 54–69, https://doi.org/10.1016/j.epsl.2012.06.050, 2012.
Nowaczyk, N. R., Liu, J., Plessen, B., Wegwerth, A., and Arz, H. W.: A High-Resolution Paleosecular Variation Record for Marine Isotope Stage 6 From Southeastern Black Sea Sediments, J. Geophys. Res.-Sol. Ea., 126, e2020JB021350, https://doi.org/10.1029/2020JB021350, 2021.
Orcutt, B. N., LaRowe, D. E., Lloyd, K. G., Mills, H., Orsi, W., Reese, B. K., Sauvage, J., Huber, J. A., and Amend, J.: IODP Deep Biosphere Research Workshop report – a synthesis of recent investigations, and discussion of new research questions and drilling targets, Sci. Dril., 17, 61–66, https://doi.org/10.5194/sd-17-61-2014, 2014.
Owens, J. D., Nielsen, S. G., Horner, T. J., Ostrander, C. M., and Peterson, L.: Thallium-isotopic compositions of euxinic sediments as a proxy for global manganese-oxide burial, Geochim. Cosmochim. Ac., 213, 291–307, 2017.
Palcu, D. V., Patina, I. S., Sandric, I., Lazarev, S., Vasiliev, I., Stoica, M., and Krijgsman, W.: Late Miocene megalake regressions in Eurasia, Sci. Rep., 11, 1–12, https://doi.org/10.1038/s41598-021-91001-z, 2021.
Popov, S. V., Shcherba, I. G., Ilyina, L. B., Nevesskaya, L. A., Paramonova, N. P., Khondkarian, S. O., and Magyar, I.: Late Miocene to Pliocene palaeogeography of the Paratethys and its relation to the Mediterranean, Palaeogeogr. Palaeoclimatol. Palaeoecol., 238, 91–106, https://doi.org/10.1016/j.palaeo.2006.03.020, 2006.
Popov, S. V., Rostovtseva, Y. V., Fillippova, N. Y., Golovina, L. A., Radionova, E. P., Goncharova, I. A., Vernyhorova, Y. V., Dykan, N. I., Pinchuk, T. N., Iljina, L. B., Koromyslova, A. V., Kozyrenko, T. M., Nikolaeva, I. A., and Viskova, L. A.: Paleontology and Stratigraphy of the Middle–Upper Miocene of the Taman Peninsula: Part 1. Description of Key Sections and Benthic Fossil Groups, Paleontol. J., 50, 1–168, 2016.
Poulton, S. W. and Canfield, D. E.: Development of a sequential extraction procedure for iron: Implications for iron partitioning in continentally derived particulates, Chem. Geol., 214, 209–221, https://doi.org/10.1016/j.chemgeo.2004.09.003, 2005.
Riedinger, N., Formolo, M. J., Lyons, T. W., Henkel, S., Beck, A., and Kasten, S.: An inorganic geochemical argument for coupled anaerobic oxidation of methane and iron reduction in marine sediments, Geobiology, 12, 172–181, 2014.
Rodriguez, M., Sakellariou, D., Gorini, C., Chamot-Rooke, N., d'Acremont, E., Nercessian, A., Tsampouraki Kraounaki, K., Oregioni, D., Delescluse, M., and Janin, A.: Seismic profiles across the North Anatolian Fault in the Aegean Sea, Geophys. Res. Abstr., Vol. 20, EGU2018-7426, EGU General Assembly 2018.
Roether, W., Beniamino, B. M., Klein, B., Bregant, D., Georgopoulos, D., Beitzel, V., Kovačević, V., Luchetta, A., Roether, W., Manca, B. B., Klein, B., Bregant, D., Georgopoulos, D., and Beitzel, V.: Recent Changes in Eastern Mediterranean Deep Waters, Science, 271, 333–335, 1996.
Rohling, E. J., Foster, G. L., Grant, K. M., Marino, G., Roberts, A. P., Tamisiea, M. E., and Williams, F.: Sea-level and deep-sea-temperature variability over the past 5.3 million years, Nature, 508, 477–482, https://doi.org/10.1038/nature13230, 2014.
Rohling, E. J., Marino, G., and Grant, K. M.: Mediterranean climate and oceanography, and the periodic development of anoxic events (sapropels), Earth-Science Rev., 143, 62–97, https://doi.org/10.1016/j.earscirev.2015.01.008, 2015.
Roveri, M., Flecker, R., Krijgsman, W., Lofi, J., Lugli, S., Manzi, V., Sierro, F. J., Bertini, A., Camerlenghi, A., De Lange, G., Govers, R., Hilgen, F. J., Hübscher, C., Meijer, P. T., and Stoica, M.: The Messinian Salinity Crisis: Past and future of a great challenge for marine sciences, Mar. Geol., 352, 25–58, https://doi.org/10.1016/j.margeo.2014.02.002, 2014.
Royden, L. H. and Papanikolaou, D. J.: Slab segmentation and late Cenozoic disruption of the Hellenic arc, Geochem. Geophy. Geosy., 12, Q03010, https://doi.org/10.1029/2010GC003280, 2011.
Rybkina, A. I., Kern, A. N., and Rostovtseva, Y. V.: New evidence of the age of the lower Maeotian substage of the Eastern Paratethys based on astronomical cycles, Sediment. Geol., 330, 122–131, https://doi.org/10.1016/j.sedgeo.2015.10.003, 2015.
Sakellariou, D., Rousakis, G., Morfis, I., Panagiotopoulos, I., Ioakim, C., Trikalinou, G., Tsampouraki-Kraounaki, K., Kranis, H., and Karageorgis, A.: Deformation and kinematics at the termination of the North Anatolian Fault: the North Aegean Trough horsetail structure, Proc. 9th Int. INQUA Meet. Paleoseismology, Act. Tectonics Archeoseismology, 237–240, 25–27 June 2018, Possidi, Greece, 2018.
Sakellariou, D. and Tsampouraki-Kraounaki, K.: Plio-Quaternary extension and strike-slip tectonics in the Aegean, in: Transform Plate Boundaries and Fracture Zones, edited by: Duarte, J., Elsevier, 339–374, https://doi.org/10.1016/B978-0-12-812064-4.00014-1, 2018.
Schmidt, J. M., Royalty, T. M., Lloyd, K. G., and Steen, A. D.: Potential Activities and Long Lifetimes of Organic Carbon-Degrading Extracellular Enzymes in Deep Subsurface Sediments of the Baltic Sea, Front. Microbiol., 12, 702015, https://doi.org/10.3389/fmicb.2021.702015, 2021.
Schrader, H. J.: Quaternary through Neogene history of the Black Sea, deduced from the paleoecology of diatoms, silicoflagellates, ebridians, and chrysomonads, in: Initial Reports of the Deep Sea Drilling Project, edited by: Ross, D. A. and Neprochnov, Y. P., Vol. 42, Part 2, U.S. Government Printing Office, Washington, 1978.
Scott, C. and Lyons, T. W.: Contrasting molybdenum cycling and isotopic properties in euxinic versus non-euxinic sediments and sedimentary rocks: Refining the paleoproxies, Chem. Geol., 324, 19–27, 2012.
Şengör, A. M. C.: The North Anatolian transform fault: its age, onset and tectonic significance, J. Geol. Soc. London, 136, 269–282, 1979.
Şengör, A. M. C., Tüysüz, O., İmren, C., Sakınç, M., Eyidoğan, H., Görür, N., Le Pichon, X., and Claude Rangin, C.: The North Anatolian Fault. A new look, Annu. Rev. Earth Planet. Sci., 33, 1–75, 2005.
Sinninge Damsté, J. S., Wakeham, S. G., Kohnen, M. E., Hayes, J. M., and de Leeuw, J. W.: A 6,000-year sedimentary molecular record of chemocline excursions in the Black Sea, Nature, 362, 827–829, 1993.
Sorlien, C. C., Akhun, S. D., Seeber, L., Steckler, M. S., Shillington, D. J., Kurt, H., Çifçi, G., Poyraz, D. T., Gürçay, S., Dondurur, D., Küçük, H. M., and Diebold, J. B.: Uniform basin growth over the last 500ka, North Anatolian Fault, Marmara Sea, Turkey, Tectonophysics, 518–521, 1–16, https://doi.org/10.1016/j.tecto.2011.10.006, 2012.
Soulet, G., Delaygue, G., Vallet-Coulomb, C., Böttcher, M. E., Sonzogni, C., Lericolais, G., and Bard, E.: Glacial hydrologic conditions in the Black Sea reconstructed using geochemical pore water profiles, Earth Planet. Sci. Lett., 296, 57–66, https://doi.org/10.1016/j.epsl.2010.04.045, 2010.
Sperling, M., Schmiedl, G., Hemleben, C., Emeis, K. C., Erlenkeuser, H., and Grootes, P. M.: Black Sea impact on the formation of eastern Mediterranean sapropel S1? Evidence from the Marmara Sea, Palaeogeogr. Palaeoclimatol. Palaeoecol., 190, 9–21, https://doi.org/10.1016/S0031-0182(02)00596-5, 2003.
Stoica, M., Krijgsman, W., Fortuin, A., and Gliozzi, E.: Paratethyan ostracods in the Spanish Lago-Mare: More evidence for interbasinal exchange at high Mediterranean sea level, Palaeogeogr. Palaeoclimatol. Palaeoecol., 441, 854–870, https://doi.org/10.1016/j.palaeo.2015.10.034, 2016.
Tari, G., Fallah, M., Kosi, W., Floodpage, J., Baur, J., Bati, Z., and Sipahioğlu, N. O.: Is the impact of the Messinian Salinity Crisis in the Black Sea comparable to that of the Mediterranean?, Mar. Pet. Geol., 66, 135–148, https://doi.org/10.1016/j.marpetgeo.2015.03.021, 2015.
Tari, G., Fallah, M., Schell, C., Kosi, W., Bati, Z., Sipahioğlu, N. Ö., Krezsek, C., Schleder, Z., Kozhuharov, E., and Kitchka, A.:. Why are there no Messinian evaporites in the Black Sea?, Pet. Geosci., 22, 381–391, 2016.
Taymaz, T., Jackson, J., and McKenzie, D.: Active tectonics of the North and Central Aegean Sea, Geophys. J. Int., 106, 433–490, 1991.
Thunell, R. C., Locke, S. M., and Williams, D. F.: Glacio-eustatic sealevel control on Red Sea salinity, Nature, 334, 601–604, 1988.
Tishchenko, I., Tari, G., Fallah, M., and Floodpage, J.: Submarine landslide origin of a tsunami at the Black Sea coast: Evidence based on swath bathymetry and 3D seismic reflection data, Interpretation, 0, SB67-BS78, 2021.
Van Baak, C. G. C., Radionova, E. P., Golovina, L. A., Raffi, I., Kuiper, K. F., Vasiliev, I., and Krijgsman, W.: Messinian events in the Black Sea, Terra Nov., 27, 433–441, https://doi.org/10.1111/ter.12177, 2015.
Van Baak, C. G. C., Vasiliev, I., Palcu, D. V, Dekkers, M. J., and Krijgsman, W.: A Greigite-Based Magnetostratigraphic Time Frame for the Late Miocene to Recent DSDP Leg 42B Cores from the Black Sea, Front. Earth Sci., 4, 1–18, https://doi.org/10.3389/feart.2016.00060, 2016.
Vasiliev, I., Reichart, G.-J., and Krijgsman, W.: Impact of the Messinian Salinity Crisis on Black Sea hydrology—Insights from hydrogen isotopes analysis on biomarkers, Earth Planet. Sci. Lett., 362, 272–282, https://doi.org/10.1016/j.epsl.2012.11.038, 2013.
Vasiliev, I., Reichart, G.-J., Grothe, A., Sinninghe Damsté, J. S., Krijgsman, W., Sangiorgi, F., Weijers, J. W. H., and van Roij, L.: Recurrent phases of drought in the upper Miocene of the Black Sea region, Palaeogeogr. Palaeoclimatol. Palaeoecol., 423, 18–31, https://doi.org/10.1016/j.palaeo.2015.01.020, 2015.
Vasiliev, I., Reichart, G.-J., Krijgsman, W., and Mulch, A.: Black Sea rivers capture significant change in catchment-wide mean annual temperature and soil pH during the Miocene-to-Pliocene transition, Glob. Planet. Change, 172, 428–439, https://doi.org/10.1016/j.gloplacha.2018.10.016, 2019.
Vasiliev, I., Feurdean, A., Reichart, G. J., and Mulch, A.: Late Miocene intensification of continentality in the Black Sea region, Int. J. Earth Sci., 109, 831–846, https://doi.org/10.1007/s00531-020-01832-w, 2020.
Wegwerth, A., Dellwig, O., Kaiser, J., Ménot, G., Bard, E., Shumilovskikh, L., Schnetger, B., Kleinhanns, I. C., Wille, M., and Arz, H. W.: Meltwater events and the Mediterranean reconnection at the Saalian–Eemian transition in the Black Sea, Earth Planet. Sci. Lett., 404, 124–135, 2014.
Wegwerth, A., Dellwig, O., Wulf, S., Plessen, B., Kleinhanns, I. C., Nowaczyk, N. R., Jiabo, L., and Arz, H. W.: Major hydrological shifts in the Black Sea “Lake” in response to ice sheet collapses during MIS 6 (130–184 ka BP), Quat. Sci. Rev., 219, 126–144, https://doi.org/10.1016/j.quascirev.2019.07.008, 2019.
Wegwerth, A., Kaiser, J., Dellwig, O., and Arz, H. W.: Impact of Eurasian Ice Sheet and North Atlantic Climate Dynamics on Black Sea Temperature Variability During the Penultimate Glacial (MIS 6, 130–184 ka BP), Paleoceanogr. Paleocl., 35, e2020PA003882, https://doi.org/10.1029/2020PA003882, 2020.
Yakushev, E. V., Chasovnikov, V. K., Murray, J. W., Pakhomova, S. V., Podymov, O. I., and Stunzhas, P. A.: Vertical hydrochemical structure of the black sea, Handb. Environ. Chem., Vol. 5 Water Pollut., 5 Q, 277–307, https://doi.org/10.1007/698_5_088, 2008.
Zinke, L. A., Mullis, M. M., Bird, J. T., Marshall, I. P. G., Jørgensen, B. B., Lloyd, K. G., Amend, J. P., and Kiel Reese, B.: Thriving or surviving? Evaluating active microbial guilds in Baltic Sea sediment, Environ. Microbiol. Rep., 9, 528–536, https://doi.org/10.1111/1758-2229.12578, 2017.
- Abstract
- Introduction
- Gateway impact on the Mediterranean and Black Sea
- BlackGate MagellanPlus workshop
- BlackGate preliminary proposal
- Conceptual drilling and coring strategy
- Site descriptions
- Code and data availability
- Team list
- Author contributions
- Competing interests
- Disclaimer
- Acknowledgements
- Financial support
- Review statement
- References
- Abstract
- Introduction
- Gateway impact on the Mediterranean and Black Sea
- BlackGate MagellanPlus workshop
- BlackGate preliminary proposal
- Conceptual drilling and coring strategy
- Site descriptions
- Code and data availability
- Team list
- Author contributions
- Competing interests
- Disclaimer
- Acknowledgements
- Financial support
- Review statement
- References