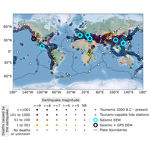
MagellanPlus Workshop: mission-specific platform approaches to assessing natural hazards that impact society
João C. Duarte
Ake Fagereng
Raphaël Paris
Patricia Persaud
Ángela María Gómez-García
Oceanic natural hazards pose threats to coastal communities worldwide. These include earthquakes, tsunamis, submarine landslide, volcanic eruptions, and tropical cyclones. Scientific ocean drilling can contribute to our understanding and assessment of these hazards through rapid response measurements of hazardous events, learning from past hazard records, and sub-seafloor monitoring and observation. With the impending retirement of the D/V JOIDES Resolution and operational limitations of the D/V Chikyu, it is important to consider other options for achieving scientific ocean drilling goals. We convened a workshop in Lisbon, Portugal, in July 2022 to identify locations where natural hazards, or preferably several different hazards, can be addressed with mission-specific platform (MSP) drilling, with a consideration of further location-based workshops. Participants split into three working groups to develop hypotheses surrounding climate and tropical cyclones, slope failure, and processes at active margins that can be tested with MSP drilling and can be addressed using the unique capabilities of these platforms. We produced 13 questions or hypotheses with recommendations on specific areas or locations for drilling. Our hope is that the results of this workshop will lay the groundwork for future pre-proposals.
- Article
(2576 KB) - Full-text XML
- BibTeX
- EndNote
Natural hazards associated with the ocean, including earthquakes, tsunamis, submarine landslides, volcanic eruptions, and tropical cyclones, can have a direct impact on coastal populations and even affect populations located far away from the coast (Koppers and Coggon, 2020). These hazards may interact, such as when tsunamis result in major direct damage and loss of life and also trigger submarine landslides, which themselves can produce tsunamis and damage subsea infrastructure like communications cables, oil and gas pipelines, and offshore wind turbines. In addition to these events, sea level rise and warming sea temperatures are resulting in more damaging tropical cyclones (Walsh et al., 2016), severe and nuisance coastal flooding (Morris and Renken, 2020; Vega et al., 2021), and larger-scale disruptions to ocean and atmospheric circulation (Caesar et al., 2018). Tectonically and climatically driven hazards operate and interact over timescales that are societally relevant, from seasonal to decadal (Telesca, 2007), and their records are preserved in the geological record. Natural hazards can be investigated in locations threatened by only one hazard, or locations where several different hazards are present. While earthquakes and associated tsunamis more commonly originate on active margins, tsunamis triggered by submarine landslides can occur on oceanic islands and passive margins as well (Fig. 1). Submarine landslides can themselves be triggered by earthquakes even on passive margins (Schulten et al., 2019) or through cyclic loading by large waves during tropical cyclones, which primarily affect the tropics but also the European Atlantic coast (Sainsbury et al., 2020). The prevalence of various hazards throughout geological history could be investigated as well as linkages with longer-term trends in climate, ocean circulation, and tectonics. The paucity of warning systems for earthquakes and tsunamis (Fig. 1) demonstrates a particular opportunity for devising monitoring networks in other areas with a high natural hazard prevalence.
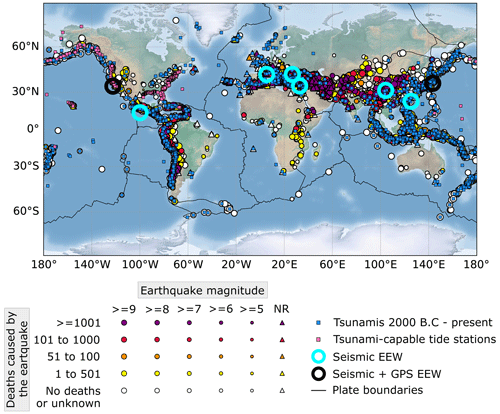
Figure 1Historic earthquakes 2150 BCE–present (NGDC/WDS, 2023a), along with tsunami events 2000 BCE–present (NGCD/WDS, 2023b), tsunami-capable tide stations (NOS/CO-OPS, 2023), and earthquake early warning (EEW) systems (Minson et al., 2015). Earthquakes shown meet at least one of the following criteria: caused at least USD 1 million in damage, resulted in 10 or more deaths, had a magnitude of 7.5 or more, had a Modified Mercalli intensity of X or greater, or generated a tsunami.
The International Ocean Discovery Program (IODP) 2050 Science Framework (Koppers and Coggon, 2020) lists investigating natural hazards impacting society as a strategic objective, with rapid response measurements of hazardous events, learning from past hazard records, and sub-seafloor monitoring and observation identified as areas where scientific ocean drilling can contribute to understanding. Assessing earthquake and tsunami hazards is specifically identified as a flagship initiative in the Framework, while climate-related hazards fall under the flagship initiative to ground-truth future climate change. Mission-specific platforms (MSPs) can provide a significant advantage over large drill ships in investigating natural hazards as they can potentially operate in shallower waters, in more restricted environments, or in sea ice; as MSPs can be specially tailored for deployment or monitoring of instrumentation; and as they have the potential for more rapid deployment in response to new events or repeat deployment over months or years to visit monitoring stations. They do have drawbacks as well, including limitations on water and coring depths as well as a restricted ability to perform shipboard analyses. MSPs are also usually smaller than the IODP vessels but are always able to carry out the minimum of IODP measurements. MSPs therefore have promise for advancing scientific ocean drilling, but the circumstances in which they can be used require definition.
MSPs are chosen by IODP to fulfill specific scientific objectives in certain conditions where the use of IODP vessels (D/V JOIDES Resolution or D/V Chikyu) is not appropriate. Whereas the JOIDES Resolution and Chikyu are dedicated drilling vessels, MSPs will be adapted to suit IODP needs as far as possible. Approaches for sample recovery of past MSPs include the use of wireline coring from geotechnical vessels or mining rigs on liftboats and of giant piston corer or seabed drills deployed from research vessels. There also exists the possibility of installing borehole observatories, though this has not been tested on MSPs to date. The ECORD Science Operator (ESO) typically provides the necessary equipment for core curation and laboratory measurements onboard, usually in containerized facilities that are placed on deck for the length of the cruise. Overall, the science party for an MSP expedition is oftentimes smaller than on an IODP vessel and needs to be more flexible, as scheduling is more variable due to contracting of MSP vessels. After an MSP expedition, the majority of sampling and analysis takes place at the Onshore Science Party (OSP) Facility in Bremen, Germany, attended by the full science party.
MSP expeditions also aim at collecting in situ downhole logs. There are multiple approaches to collect downhole logs, with the standard one for MSP (and the JOIDES Resolution) being wireline logging, where tools are sent downhole connected to a cable, a winch, and acquisition equipment. Wireline logging allows real-time data acquisition against depth. The datasets acquired with this method on MSP expeditions can include borehole diameter, total and spectral gamma rays, formation resistivity or conductivity, magnetic susceptibility, acoustic velocity, borehole fluid properties, and borehole wall images. As with the MSP expeditions' flexibility for drilling platform and strategy, the logging equipment can be adapted to various operational setting and scientific objectives. For example, the European Petrophysics Consortium (EPC), part of ESO, has a set of wireline logging tools referred to as “slimline” and designed by ALT, Mount Sopris, Antares, and Geovista. These tools are relatively short (< 2.5 m), lightweight (< 20 kg), and narrow (4–6 cm diameter), and they can be run downhole as stand-alone sensors or combined into toolstrings of up to four tools and less than 40 kg. ESO–EPC provided wireline logging services on five MSP expeditions (see the methods section in Camoin et al., 2007; Mountain et al., 2010; Webster et al., 2011; Morgan et al., 2017; McNeill et al., 2019). Wireline logging services have also been provided by other service companies (see the methods section in Backman et al., 2006; Andrén et al., 2015). ESO also deployed logging-while-tripping slimline tools from seabed drills during Expedition 357 (see the methods section in Früh-Green et al., 2017).
With the impending retirement of the D/V JOIDES Resolution and operational limitations of the D/V Chikyu, it is important to consider other options for ocean drilling research. To explore the potential to use MSPs to address natural hazards impacting society, we convened a workshop in Lisbon, Portugal, in July 2022 that brought together 28 researchers from around the world, including nine early-career researchers, both in person and virtually (Daigle et al., 2022).
The objectives of the workshop were (1) to form working groups that would develop plans to address key scientific questions discussed at the meeting, (2) to identify locations where tsunamis, submarine landslides, volcanic eruptions, and tropical cyclones, or preferably several of these hazards, can be addressed with MSP drilling, with a consideration of further location-based workshops, and (3) to develop a set of hypotheses that can be tested with MSP drilling which would lay the groundwork for future pre-proposals.
During the workshop, we created three working groups that developed hypotheses and questions focused on three main topics: climate and tropical cyclones, slope failure, and processes at active margins. These hypotheses and questions can be used to develop drilling proposals for specific locations. Specific locations mentioned by the working groups are shown in Fig. 2.
3.1 Working Group 1: climate and tropical cyclones
The climate and tropical cyclones working group agreed that the northern Gulf of Mexico presents the best location to address questions about climate change and its influence on tropical cyclone frequency and intensity. The Gulf of Mexico is ideal because of its overall thick sediment column (up to 20 km; Galloway, 2008) and well-preserved sedimentary sections in salt withdrawal minibasins, growth faults, river deltas, and basin-floor fans (Martin, 1978). The group phrased two hypotheses of global significance that may be addressed in the Gulf of Mexico.
3.1.1 Hypothesis 1
We hypothesize that past hurricane frequency and intensity in the Gulf of Mexico have responded to changes in the strength of ocean circulation patterns, most notably the strength of the Gulf Stream and the Atlantic Meridional Overturning Circulation (AMOC). Warm core eddies spun off the Loop Current can cause rapid intensification of hurricanes (e.g., Hurricane Katrina in 2005; Scharroo et al., 2005). The reconstruction of sea surface temperatures (SSTs) and storm frequency from sedimentary deposits in the Gulf of Mexico could be used to understand how much influence the variability in the Gulf Stream dynamics has on Gulf of Mexico storms.
Drill site needs and potential outcomes. MSPs could be used to collect very high-resolution storm and SST records in areas with continuous late Pleistocene to Holocene sedimentary sections. These time intervals contain many episodes of AMOC slowdown (Heinrich Events, Younger Dryas) that would allow us to understand the impact of AMOC variability on hurricane development in this region.
Potential drill sites. Salt withdrawal minibasins along the Gulf of Mexico's margin contain suitable deposits, typically representing a sediment thickness of 100–200 m.
3.1.2 Hypothesis 2
Storm frequency has a strong influence on precipitation and streamflow in the western Gulf of Mexico and southwestern United States. Hurricane Harvey (2017) dropped more than 1500 mm of rain in parts of southeast Texas in less than a week and storm-related precipitation is expected to increase as climate warms over the 21st century (Bacmeister et al., 2018). Cores from the Gulf of Mexico have provided evidence for past occurrence of large flood events on the Mississippi River (e.g., deglacial meltwater pulses; Clark et al., 1996; Brown and Kennett, 1998).
Drill site needs and potential outcomes. Drill sites should be located offshore of rivers that provide sedimentary flux from catchments that are affected by tropical cyclones. These locations can provide information on flooding events, particularly those during past climate intervals warmer than the present day such as the mid-Holocene or previous interglacials. MSP drilling could include long cores, which could be combined with borehole or seafloor monitoring of the development of mud flow events to establish modern depositional system analogs and seismic surveys of the continental shelf to map potential sediment source areas.
Potential drill sites. Suitable locations can be found offshore of the mouths of the Rio Grande, Colorado River, Brazos River, Sabine River, and other rivers on the western Gulf of Mexico coast. Salt withdrawal minibasins offshore of the mouth of the Rio Grande may make the best locations as they tend to preserve more continuous sedimentary records.
3.2 Working Group 2: submarine slope failure
3.2.1 Hypothesis 1: preconditioning factors cause repeat landslides
We hypothesize that through the Neogene, the presence of similar preconditioning factors has typically caused submarine landslides to occur repeatedly at the same location. Preconditioning factors can include sediment properties, pore fluid pressure, fluid chemistry, mechanical stratigraphy, and oversteepening/slope angle (Hampton et al., 1996). These can be investigated with hydraulic piston cores (equivalent to the JOIDES Resolution advanced piston coring (APC) tool) down to 1000 m water depth in most cases. Required measurements include core physical properties and pore fluid chemistry, in situ pore pressure and heat flow measurements, and anything that can provide evidence of changes in stresses, e.g., borehole breakouts or drilling-induced tensile fractures obtained from multi-axis caliper or borehole image logs. In situ observatories can help establish trends in pore pressure, heat flow, and stress over time.
Drill site needs and potential outcomes. Drill sites should be located near the headscarp of a submarine landslide. Core analysis, downhole measurements, and in situ observatories will provide evidence of the presence or absence of preconditioning factors, and how these change over time.
Potential drill sites. There are many locations that could be targeted on both active and passive margins. The Cape Fear Slide, Storegga Slide, and Sahara Slide are potential targets on passive margins, while active margins such as the Nankai Trough, the Sumatra Margin, and the Hikurangi Margin could be investigated.
3.2.2 Hypothesis 2: landslides are externally triggered
Preconditioning factors are necessary but not sufficient for submarine landslides, and in most cases an external trigger is needed. Triggers include earthquakes, storm wave loading, abrupt shifts in sedimentation, tectonic oversteepening, changes in ocean temperature, gas hydrate dissociation, volcanism, and salt diapirism (e.g., Urlaub et al., 2013). This hypothesis can be tested by recovering cores, fluid samples, and pore pressures to demonstrate that slope failure could occur in an area that would otherwise be stable. Note that, if hypothesis 1 is true, then one initiation can cause the area to be prone to failure for many years afterwards.
Drill site needs and potential outcomes. Ideal sites to test this hypothesis should be locations with no evidence of slope failure but near known submarine landslides. Measured properties could be used as inputs for models to predict conditions for slope failure.
Potential drill sites. This hypothesis could be tested at the same locations as the previous hypothesis, with the addition of volcanic islands such as the Azores.
3.2.3 Hypothesis 3: retrogressive failure leaves a signature
Retrogressive failure leaves a detectable perturbation of pore pressure and heat flow. The pore pressure perturbation is related to failure propagation upslope, and the heat flow perturbation is a leftover signature of it. Anomalously high heat flow also could be evidence of salt diapirism. This technique can also be used to infer gas hydrate (in)stability. This hypothesis would best be tested with in situ measurements and longer-term monitoring with dense spatial sampling. One particular location that should be targeted is the distal toe of a submarine landslide, although this may be deeper than MSP capabilities (∼ 1700 m) in most locations. For example, the toe of the Cape Fear Slide is at about 5.4 km water depth (Popenoe et al., 1993), while that of the Storegga Slide is at 3–3.5 km water depth (Bugge et al., 1988).
Drill site needs and potential outcomes. Potential sites should be located at submarine landslides with known retrogressive failure. Long-term measurements of pore pressure and heat flow can provide evidence of the current state of the subsurface around submarine landslides and its response to external perturbations.
Potential drill sites. The Cape Fear Slide is a well-studied example of retrogressive failure that could be targeted to test this hypothesis.
3.2.4 Hypothesis 4: active margins have more earthquakes than landslides
Submarine landslides occur less frequently than earthquakes on active margins because of (a) seismic strengthening and (b) sediment accumulation rates. It is established that seismic shaking tends to increase the shear strength of sediments (Sawyer and DeVore, 2015), so slope failure on an active margin would tend to require a large amount of sediment to accumulate between earthquakes.
Drill site needs and potential outcomes. To test this hypothesis, high-resolution seismic data, core data to understand strength and sedimentation rate, and a good paleo-seismological record are required. A drilling strategy to test this hypothesis would consist of a transect across an accretionary prism with perched basins that preserved mass transport deposits.
Potential drill sites. The Nankai Trough or the Hikurangi Margin make excellent candidates to test this hypothesis due to the large volume of existing data.
3.3 Working Group 3: active margins
3.3.1 Hypothesis 1: major earthquakes have precursors
Transient events are observed at plate boundaries worldwide, but we currently do not understand how these relate to the timing of larger earthquakes. In a few cases, slow slip events at subduction zones have been observed in the weeks to months prior to great megathrust earthquakes (e.g., the Tōhoku-oki 2011 and Iquique 2014 earthquakes) (Ito et al., 2013; Ruiz et al., 2014). Resolving whether great earthquakes have precursors is a societally important question, critical for more effective, short-term earthquake forecasting. As most subduction zone earthquakes nucleate on offshore megathrusts, detailed offshore monitoring of potential transient deformation is required.
Drill site needs and potential outcomes. Drill sites should be located near active faults in areas with large earthquakes. Due to the high-noise ocean environment, the most viable tool to undertake this type of monitoring is borehole observatories, specifically detecting volumetric strain using changes in formation pore pressure as a proxy, as done in boreholes offshore subduction margins in Costa Rica, Japan, and New Zealand (Davis et al., 2015; Araki et al., 2017; Wallace et al., 2019).
Potential drill sites. Highly active transform faults that are the site of frequently recurring moderate to large-magnitude earthquakes, such as the Gofar transform fault with quasi-periodic Mw 6–7 events every 5–6 years (McGuire, 2008), could be ideal targets to investigate potential precursors through the installation of borehole observatories. Amphibious experiments complementing the already existing infrastructure could be developed in both the Chilean Margin (Barrientos and the National Seismological Center (CSN) Team, 2018) and the Marmara Sea (MARSite; Özel et al., 2017). The Salton Sea and the Gulf of California may also be potential sites, as a southward extension of the U.S. Geological Survey Parkfield, CA, network, but are complicated by transtensional deformation (Brothers et al., 2009). In addition, the Hikurangi Margin, Hellenic Arc, and Southwest Iberian Margin could be other locations targeted to test this hypothesis.
3.3.2 Hypothesis 2: rupture barriers are persistent
Several hypotheses exist to explain rupture terminations that appear to be persistent over the timescale of available earthquake records. These range from the presence of plate boundary transitions (Wallace et al., 2012), to changes in upper-plate/lower-plate properties and/or geometrical changes (Collot et al., 2004), stress heterogeneity (Huang, 2018), and the presence of positive relief structures such as seamounts or ridges (Sparkes et al., 2010). However, no conclusive evidence exists to confirm that these historical or recent rupture terminations are persistent over longer timescales. Potential boundary-breaking earthquake ruptures could lead to earthquakes that substantially exceed historical or instrumentally observed magnitudes (maybe even M > 10) and that have recurrence intervals of several thousands of years or more (Goldfinger et al., 2013). This is much longer than the current length of available paleoseismic records along any subduction zone around the world, meaning we cannot reliably exclude their occurrence.
To evaluate the persistence of rupture/segment barriers and unveil (or discard) the existence of these larger-than-observed earthquakes, the available paleoseismic records need to be extended back in time. Long-term paleoseismic records are thus needed along the entire length of subduction margins. This requires sedimentary records in both the onshore (coastal and lake) and offshore realm and relies on the identification of secondary seismic effects such as shaking imprints (e.g., turbidites, soft-sediment deformation) and tsunami deposits. Identification of synchronous deposits along an entire subduction margin could hint at these extreme events, but the current limitations of dating accuracy do not allow distinguishing single, large events from short-term successions of ruptures (e.g., stress triggering). Therefore, key records at locations that are currently believed to form rupture barriers are essential to verify the existence of imprint stacks, resulting from rupture cascades, while the presence of a single deposit could hint at large, through-going ruptures.
Drill site needs and potential outcomes. Drill sites need to have historic earthquake records that show earthquake boundaries; a detailed understanding of sediment routing systems and several preferentially isolated depositional basins spanning the entire margin and containing high-resolution, continuous paleoseismic event deposit stratigraphies, for which sedimentary source areas can be well constrained to allow for the reconstruction of past rupture areas; and a straight margin to avoid complications of changes in fault geometry (unless testing the role of plate margin geometry on arresting earthquake rupture is desired). The outcome of drilling would ideally be a long-term extension of the paleoseismic record.
Potential drill sites. The South American subduction zone is a potential target to investigate this hypothesis. It generated the largest known earthquake (magnitude 9.6, in 1960), and it is potentially able to generate the largest earthquakes of any subduction zone (Graham et al., 2021). In addition, many lakes along the margin could be sampled by continental drilling to constrain the paleoseismic record (Bernhardt et al., 2015). Other potential locations include the Marmara Sea, the Salton Sea/Gulf of California, the Hikurangi Margin, Cascadia, the Hellenic Arc, and the Calabrian Arc.
3.3.3 Hypothesis 3: fault coupling characteristics are persistent in space and time
Subduction plate interfaces commonly have spatially variable interseismic coupling, where strongly coupled segments are most likely to produce large earthquakes and weakly coupled segments tend to slip aseismically (Fagereng, 2011; Saito and Noda, 2022). It is unclear whether geodetically constrained coupling patterns persist over multiple earthquake cycles and also to what extent poorly coupled regions may slip during earthquakes that nucleate in adjacent coupled areas. Controls on coupling could include fluid pressure (Moreno et al., 2014), downgoing plate roughness (Ruff, 1989; Wang and Bilek, 2011; van Rijsingen et al., 2018), or rock physical properties at depth, including grain size, mineralogy, and fluid composition (Chen et al., 2013; Scholz, 2019).
Drill site needs and potential outcomes. Direct evidence for temporal variation in locking may be sought from the paleoseismic record by looking for evidence for past earthquakes and how their spatial pattern compares with current geodetic locking. This would require a margin where the current geodetic locking pattern is well characterized and where there are appropriate sites for paleo-seismicity (e.g., Chilean margin). The expectation, if coupling characteristics are persistent in time and space, would be that in locked regions, there is evidence for large earthquakes (where the meaning of “large” should be calculated from the size of the locked patch). These earthquakes should, however, not have propagated into poorly coupled segments. Another direct measure is variations in interseismic periods if observatories can be installed and maintained over multiple earthquake cycles.
Potential drill sites. Sites with existing reflection seismic and other data that could be used to test this hypothesis include the Chilean Margin, the Marmara Sea, the Hellenic Arc, the Salton Sea/Gulf of California, Cascadia, the Nankai Trough, the Hikurangi Margin, and Costa Rica Margin.
3.3.4 Hypothesis 4: subduction earthquakes are cyclic at multiple timescales
The 2011 Mw 9.0 Tōhoku-oki Japan earthquake occurred in an area where scientists thought large-magnitude earthquakes were less likely (Stein et al., 2012). In contrast, the 2010 Mw 8.8 Maule earthquake in Chile was somewhat expected but extended beyond the boundaries of the seismic gap that was believed to be present (Métois et al., 2012). These recent, very high-magnitude earthquakes painfully highlight how little we still know about megathrust earthquake recurrence along subduction zones, despite recent advances and widespread paleo-seismological studies. The concepts of seismic gaps and characteristic earthquakes are simplified and need further refining.
Mapping the spatiotemporal behavior of megathrust earthquakes thus forms a crucial step towards validation of physics-based earthquake cycle models but is currently not possible on sufficiently long timescales and/or spatial extents along any of the subduction zones. Instrumental and historical records are too short, and coastal records (tsunami deposits, uplifted terraces or corals, subsided paleosols) are affected by global eustatic sea level change and do not extend far beyond the last maximum sea level high stand of the Holocene.
Drill site needs and potential outcomes. Scientific ocean (and continental) drilling and coring of high-resolution marine and/or lacustrine paleoseismic archives extending well into the late Pleistocene and further back in time are the only reasonable approach to potentially deliver observational data on timescales long enough to robustly test the earthquake (super-)cycle hypothesis. Therefore, drill sites need to have well-preserved, continuous sedimentary sections.
Potential drill sites. Drilling to test this hypothesis could be conducted at the Chilean Margin, at the Hikurangi Margin, and in Cascadia.
3.3.5 Hypothesis 5: fault slip rates vary over multiple seismic cycles
Fault slip rates are critical for seismic hazard assessment and can be calculated over a range of different timescales from < 1 year to millions of years. Variations between short-term and long-term slip rates have been recorded, bringing into question the usefulness of slip rates calculated over a particular time period for seismic hazard assessment; i.e., are geological rates relevant to apply to modern seismic hazard estimates? Do modern satellite-derived slip rates (from GPS) give a good indication of the areas most at risk from earthquakes (e.g., Bell et al., 2011; Cowie et al., 2012; Fagereng and Biggs, 2019)? Our understanding of fault growth and fault slip is least well-constrained in the 104–106-year range (reviewed in Pan et al., 2022). There are a number of ways in which we can assess slip rates in the range of 104–106 years using offshore MSP drilling/piston core data, including targeted giant piston coring or short drilled sections on either side of a fault (or one side of the fault only if horizons can be confidently correlated) to calculate offset and slip rate: using submarine paleo-seismology to identify evidence of individual earthquake slip events in high-resolution seismic data with ground-truthing of age and timing or using an onshore–offshore approach for a comparison of slip rates over different timescales on an active normal fault.
Drill site needs and potential outcomes. Potential locations to study slip rates through time would ideally have the following: (i) high sedimentation rates, (ii) high slip rates (to give the greatest chance of slip rate variations being resolved), (iii) a constrained onshore record of uplift rates to compare with results of ocean/lake drilling, and (iv) high-resolution seismic reflection data imaging basin stratigraphy in the hanging wall and ideally also in the footwall.
Potential drill sites. Drilling to test this hypothesis could be conducted at active rift zones and potentially at suitable transform zones and subduction margins, e.g.,t the Corinth Rift (Greece), the Salton Sea/Gulf of California, the Marmara Sea, the Chilean Margin, and the Hikurangi Margin.
3.3.6 Hypothesis 6: faults grow rapidly to their full length
Seismological and geodetic data reveal that earthquake rupture patterns are complex and variable, often with temporal and spatial clustering of events (e.g., Wells and Coppersmith, 1994). In contrast, ancient normal faults commonly observed in high-resolution 3D seismic reflection datasets reveal strikingly consistent patterns of displacement accumulation along strike, commonly described as the classic “bell-shaped” cumulative displacement profile with greatest overall displacement in the center of the fault decreasing toward the tips (e.g., Cowie and Scholz, 1992). Other mature faults within extended systems have consistent slip rates along strike, potentially indicating linkage of segments at depth. Exactly how faults evolve in terms of how multiple earthquake cycles and aseismic slip accrue to produce the long-term fault geometry is unknown. Most work on this problem has focused on normal faults, as they are associated with a convenient marker of fault growth in the form of sediment thickness increases in the hanging wall when a fault is active at the Earth's surface (i.e., it is a “growth fault”). Therefore, to address the question of “how faults grow” will likely require observations from active continental rifts. Understanding how faults develop has relevance to earthquake hazards because of how we interpret fault slip rates over different time periods (see Sect. 3.3.5 above) and how earthquake slip builds up to fault slip over longer timescales. One category of normal faults that is relatively understudied is outer rise normal faults at subduction zones. These faults, caused by flexure of the downbending subducting plate, are a significant tsunami hazard, and they are potential sites for investigating the normal fault evolution of this hypothesis.
Drill site needs and potential outcomes. It may be possible to investigate in detail how faults establish themselves and evolve both laterally and in terms of displacement accrual by identifying a study location where a very young fault exists at a shallow depth, which is well imaged by 3D or pseudo-3D high-resolution seismic reflection data. If age-constraints are available from drilling/piston coring, the interpretation of high-resolution seismic reflection data will allow variations in sediment thickness between the hanging wall and footwall to be investigated at the millennial scale and hence how slip has accrued along different parts of the fault. This timescale links the supra-millennial scale of modern geological and seismological observation and the million-year-averaged observations from seismic reflection data in rifts.
Potential drill sites. Drilling to test this hypothesis could be conducted at the following locations: the Corinth Rift (Greece) (McNeill et al., 2019; Nixon et al., 2016), the Whakatane Graben (New Zealand) (Taylor et al., 2004), and outer rise normal fault systems at subduction margins.
3.3.7 Hypothesis 7: tectonic activity is linked to the timing of volcanic eruptions
Active submarine volcanoes are linked to several of the deadliest geohazards such as tsunami, earthquakes, landslides, pyroclastic material, and gas release. However, they pose difficult drilling conditions as they are covered by thin microbial crusts, and they have active hydrothermal systems and unstable slopes. MSP drilling presents an ideal way to sample these locations. Volcanic activity (frequency, volume, magma flux) is affected by tectonic activity at large length scales (e.g., plate tectonics) and smaller scales (e.g., development of preferential pathways for magma ascent due to local deformation). On the other hand, tectonic activity can also be affected by volcanism, for example in the case of magma ascent triggering localized seismic activity. This interconnection can be investigated through a high-resolution record of eruptive styles at individual centers that will give a characterization of their evolution in time, their intensity, and their spatial and temporal distribution. Large tectonic events recorded in sedimentary basins, like onlap surfaces, seismogenic turbidites, and homogeneities, can correlate with activation/deactivation of different volcanic centers, changes in eruptive style, and particularly large explosive eruptions.
Drill site needs and potential outcomes. Drilling should be conducted at sites with a well-preserved record of volcanism and tectonic events. Integration of paleoseismic and tephrochronological records need a good correlation between onshore and offshore records. Offshore geodesy would also be a priority to reconstruct the evolution of deformation around and within these volcanic centers. Possible correlations also exist with the paleoclimate record.
Potential drill sites. This hypothesis could be tested by drilling at the Chilean Margin, the Kolumbo submarine volcano northeast of Santorini (building on recent IODP drilling), Etna, the Hikurangi Margin, the Hellenic Arc, and the Calabrian Arc.
In this workshop, we defined questions and hypotheses about natural hazards that could be tested specifically with MSPs. The unique characteristics of MSPs compared to IODP drill ships require some shifts in our thinking about how to interrogate the subsurface but also present opportunities in terms of long-term monitoring, drilling in shallow water, and amphibious proposals. The workshop discussions recognized the growing needs of highly populated regions along tectonically active or hazardous settings for a better assessment of earthquake, tsunami, volcanic, and climate hazards. A number of key questions/hypotheses, in combination with potential target locations and research strategies, were formulated that need to be tested in order to mitigate future risks.
MSP missions have the potential to contribute to the development of amphibious observatories, taking advantage of well-monitored onshore regions and leveraging partnerships with the continental drilling community. We considered that this strategy could be prioritized in the near future. The flexibility of an MSP and the relatively low costs in some cases make it worth the community effort in building a long-term infrastructure that allows us to monitor diverse geohazards from such observatories. The climate and tropical cyclones working group identified the Gulf of Mexico, where key questions can be answered by MSP drilling. The submarine slope failure working group identified areas on both passive and active margins where hypotheses can be tested, including the Cape Fear Slide, the Storegga Slide, the Sahara Slide; the Nankai Trough, the Sumatra Margin, and the Hikurangi Margin; and volcanic islands such as the Azores. Finally, the active margins working group selected priority sites for the near future including the Chilean margin, the Hikurangi Margin, the Marmara Sea, the Salton Sea/Gulf of California, Corinth Rift, and outer-rise normal fault systems. Other sites with high hazard exposure on active margins include regions around the Hellenic and Calabrian arcs, the Southwest Iberian, Cascadia, and the Sumatra and Hikurangi margins. We hope that this work serves as the foundation for future drilling proposals in the regions we identified or in other areas around the world.
The datasets containing the historic earthquakes 2150 BCE–present (https://doi.org/10.7289/V5TD9V7K, NGDC/WGS, 2023a), the tsunami events 2000 BCE–present (https://doi.org/10.7289/V5PN93H7, NGDC/WGS, 2023b) and the tsunami-capable tide stations (https://tidesandcurrents.noaa.gov/tsunami/, NOS/CO-OPS, 2023) are available at the corresponding websites of the NOAA National Geophysical Data Center/World Data Service.
Tiago Alves (School of Earth and Environmental Sciences, Cardiff University, Cardiff, UK), Fernando Barriga (Instituto Dom Luiz, Faculty of Sciences, University of Lisbon, Lisbon, Portugal), Rebecca Bell (Department of Earth Science and Engineering, Imperial College London, London, UK), Julia Carvalho (Department of Earth Sciences, University of Pisa, Pisa, Italy; Department of Earth Sciences, University of Florence, Florence, Italy), Yu-Chun Chang (Department of Earth and Environmental Sciences, University of Manchester, Manchester, UK), Gareth Davies (Geoscience Australia, Canberra, Australia), Davide Gamboa (Department of Geosciences, University of Aveiro, Aveiro, Portugal), Álvaro González (Centre de Recerca Matemàtica, Barcelona, Spain), Achim Kopf (MARUM, University of Bremen, Bremen, Germany), Holger Kuhlmann (MARUM, University of Bremen, Bremen, Germany), Lisa McNeill (School of Ocean and Earth Science, University of Southampton, Southampton, UK), Uisdean Nicholson (School of Energy, Geoscience, Infrastructure and Society, Heriot-Watt University, Edinburgh, UK), Paraskevi Nomikou (Faculty of Geology and Geo-Environment, University of Athens, Athens, Greece), Michael Strasser (Department of Geology, University of Innsbruck, Innsbruck, Switzerland), Mary Thompson (Department of Geology & Geophysics, Texas A&M University, College Station, TX, USA), Michael Toomey (United States Geological Survey, Reston, VA, USA), Caroline Ummenhofer (Woods Hole Oceanographic Institution, Woods Hole, MA, USA), Paola Vannucchi (Department of Earth Sciences, University of Florence, Florence, Italy), Laura Wallace (GNS New Zealand, Lower Hutt, New Zealand; Institute for Geophysics, The University of Texas at Austin, Austin, TX, USA), and Katleen Wils (Department of Geology, Ghent University, Ghent, Belgium).
HD: conceptualization, funding acquisition, investigation, writing. JD: conceptualization, funding acquisition, investigation, resources, writing. AF: conceptualization, funding acquisition, investigation, writing. RP: conceptualization, funding acquisition, investigation, writing. PP: conceptualization, funding acquisition, investigation, writing. AMGG: investigation, visualization, writing. Workshop participants: investigation, writing.
The contact author has declared that none of the authors has any competing interests.
Any use of trade, firm, or product names is for descriptive purposes only and does not imply endorsement by the U.S. Government.
Publisher’s note: Copernicus Publications remains neutral with regard to jurisdictional claims made in the text, published maps, institutional affiliations, or any other geographical representation in this paper. While Copernicus Publications makes every effort to include appropriate place names, the final responsibility lies with the authors.
The authors wish to thank the students and staff of Instituto Dom Luiz for their assistance during the workshop, in particular Célia Lee.
This workshop was funded by the European Consortium for Ocean Research Drilling (ECORD) and the International Continental Scientific Drilling Program (ICDP). Funding for participation of US-based scientists was provided by the United States Science Support Program (USSSP).
This paper was edited by Nadine Hallmann and reviewed by Ken Ikehara and one anonymous referee.
Andrén, T., Jørgensen, B. B., Cotterill, C., and the Expedition 347 Scientists: Expedition 347 summary, Proc. IODP, 347, 1–66, https://doi.org/10.2204/iodp.proc.347.101.2015, 2015.
Araki, E., Saffer, D. M., Kopf, A. J., Wallace, L. M., Kimura, T., Machida, Y., Ide, S., Davis, E., and the Expedition 365 Scientists: Recurring and triggered slow-slip events near the trench at the Nankai Trough subduction megathrust, Science, 356, 1157–1160, https://doi.org/10.1126/science.aan3120, 2017.
Backman, J., Moran, K., McInroy, D. B., Mayer, L. A., and the Expedition 302 Scientists: Expedition 302 summary, Proc. IODP, 302, 1–22, https://doi.org/10.2204/iodp.proc.302.101.2006, 2006.
Bacmeister, J. T., Reed, K. A., Hannay, C., Lawrence, P., Bates, S., Truesdale, J. E., Rosenbloom, N., and Levy, M.: Projected changes in tropical cyclone activity under future warming scenarios using a high-resolution climate model, Climatic Change, 146, 547–560, https://doi.org/10.1007/s10584-016-1750-x, 2018.
Barrientos, S. and the National Seismological Center (CSN) Team: The seismic network of Chile, Seismol. Res. Lett., 89, 467–474, https://doi.org/10.1785/0220160195, 2018.
Bell, R. E., McNeill, L. C., Henstock, T. J., and Bull, J. M.: Comparing extension on multiple time and depth scales in the Corinth Rift, Central Greece, Geophys. J. Int., 186, 463–470, https://doi.org/10.1111/j.1365-246X.2011.05077.x, 2011.
Bernhardt, A., Melnick, D., Hebbeln, D., Lückge, A., and Strecker, M. R.: Turbidite paleoseismology along the active continental margin of Chile – Feasible or not?, Quaternary Sci. Rev., 120, 71–92, https://doi.org/10.1016/j.quascirev.2015.04.001, 2015.
Brothers, D. S., Driscoll, N. W., Kent, G. M., Harding, A. J., Babcock, J. M., and Baskin, R. L.: Tectonic evolution of the Salton Sea inferred from seismic reflection data, Nat. Geosci., 2, 581–584, https://doi.org/10.1038/ngeo590, 2009.
Brown, P. A. and Kennett, J. P.: Megaflood erosion and meltwater plumbing changes during last North American deglaciation recorded in Gulf of Mexico sediments, Geology, 26, 599–602, https://doi.org/10.1130/0091-7613(1998)026<0599:MEAMPC>2.3.CO;2, 1998.
Bugge, T., Belderson, R. H., and Kenyon, N. H.: The Storegga Slide, Philos. T. Roy. Soc. A, 325, 357–388, https://doi.org/10.1098/rsta.1988.0055, 1988.
Caesar, L., Rahmstorf, S., Robinson, A., Feulner, G., and Saba, V.: Observed fingerprint of a weakening Atlantic Ocean overturning circulation, Nature, 556, 191–196, https://doi.org/10.1038/s41586-018-0006-5, 2018.
Camoin, G. F., Iryu, Y., McInroy, D. B., and the Expedition 310 Scientists: Methods, Proc. IODP, 310, 1–43, https://doi.org/10.2204/iodp.proc.310.103.2007, 2007.
Chen, W. P., Yu, C. Q., Tseng, T. L., Yang, Z., Wang, C. yuen, Ning, J., and Leonard, T.: Moho, seismogenesis, and rheology of the lithosphere, Tectonophys., 609, 491–503, https://doi.org/10.1016/j.tecto.2012.12.019, 2013.
Clark, P. U., Alley, R. B., Keigwin, L. D., Licciardi, J. M., Johnsen, S. J., and Wang, H.: Origin of the first global meltwater pulse following the Last Glacial Maximum, Paleoceanogr. Paleoclimatology, 11, 563–577, https://doi.org/10.1029/96PA01419, 1996.
Collot, J.-Y., Marcaillou, B., Sage, F., Michaud, F., Agudelo, W., Charvis, P., Graindorge, D., Gutscher, M.-A., and Spence, G.: Are rupture zone limits of great subduction earthquakes controlled by upper plate structures? Evidence from multichannel seismic reflection data acquired across the northern Ecuador–southwest Colombia margin, J. Geophys. Res.-Sol. Ea., 109, B11103, https://doi.org/10.1029/2004JB003060, 2004.
Cowie, P. A. and Scholz, C. H.: Displacement-length scaling relationship for faults: data synthesis and discussion, J. Struct. Geol., 14, 1149–1156, https://doi.org/10.1016/0191-8141(92)90066-6, 1992.
Cowie, P. A., Roberts, G. P., Bull, J. M., and Visini, F.: Relationships between fault geometry, slip rate variability and earthquake recurrence in extensional settings, Geophys. J. Int., 189, 143–160, https://doi.org/10.1111/j.1365-246X.2012.05378.x, 2012.
Daigle, H., Duarte, J., Fagereng, A., Paris, R., Persaud, P., Bell, R., Davies, G., Nomikou, P., Stewart, M., Toomey, M., Ummenhofer, C., Wallace, L., Alves, T., Carvalho, J., Chang, Y., Gamboa, D., Gómez-García, A. M., González, A., Kopf, A., Kuhlmann, H., Le Ber, E., McNeill, L., Nicholson, U., Strasser, M., Thompson, M., Vannucchi, P., and Wils, K.: MagellanPlus Workshop: Mission-specific platform approaches to assessing natural hazards that impact society, MagellanPlus workshop report, ECORD/ICDP, https://www.ecord.org/?ddownload=16319 (last access: 29 September 2023), 2022.
Davis, E. E., Villinger, H., and Sun, T.: Slow and delayed deformation and uplift of the outermost subduction prism following ETS and seismogenic slip events beneath Nicoya Peninsula, Costa Rica, Earth Planet. Sci. Lett., 410, 117–127, https://doi.org/10.1016/j.epsl.2014.11.015, 2015.
Fagereng, Å.: Wedge geometry, mechanical strength, and interseismic coupling of the Hikurangi subduction thrust, New Zealand, Tectonophysics, 507, 26–30, https://doi.org/10.1016/j.tecto.2011.05.004, 2011.
Fagereng, Å. and Biggs, J.: New perspectives on “geological strain rates” calculated from both naturally deformed and actively deforming rocks, J. Struct. Geol., 125, 100–110, https://doi.org/10.1016/j.jsg.2018.10.004, 2019.
Früh-Green, G. L., Orcutt, B. N., Green, S. L., Cotterill, C., and the Expedition 357 Scientists: Expedition 357 summary, Proc. IODP, 357, 1–34, https://doi.org/10.14379/iodp.proc.357.101.2017, 2017.
Galloway, W. E.: Depositional evolution of the Gulf of Mexico sedimentary basin, Sediment. Basins World, 5, 505–549, https://doi.org/10.1016/S1874-5997(08)00015-4, 2008.
Goldfinger, C., Ikeda, Y., Yeats, R. S. and Ren, J.: Superquakes and supercycles, Seismolog. Res. Lett., 84, 24–32, https://doi.org/10.1785/0220110135, 2013.
Graham, S. E., Loveless, J. P., and Meade, B. J.: A Global Set of Subduction Zone Earthquake Scenarios and Recurrence Intervals Inferred From Geodetically Constrained Block Models of Interseismic Coupling Distributions, Geochem. Geophys. Geosyst., 22, e2021GC009802, https://doi.org/10.1029/2021GC009802, 2021.
Hampton, M. J., Lee, H. J., and Locat, J.: Submarine landslides, Rev. Geophys., 34, 33–59, https://doi.org/10.1029/95RG03287, 1996.
Huang, Y.: Earthquake rupture in fault zones with along-strike material heterogeneity, J. Geophys. Res.-Sol. Ea., 123, 9884–9898, https://doi.org/10.1029/2018JB016354, 2018.
Ito, Y., Hino, R., Kido, M., Fujimoto, H., Osada, Y., Inazu, D., Ohta, Y., Iinuma, T., Ohzono, M., Miura, S., and Mishina, M.: Episodic slow slip events in the Japan subduction zone before the 2011 Tohoku-Oki earthquake, Tectonophys., 600, 14–26, https://doi.org/10.1016/j.tecto.2012.08.022, 2013.
Koppers, A. A. P. and Coggon, R. (Eds.): Exploring Earth by Scientific Ocean Drilling: 2050 Science Framework, International Ocean Discovery Program, College Station, TX, https://doi.org/10.6075/J0W66J9H, 2020.
Martin, R. G.: Northern and eastern Gulf of Mexico continental margin: stratigraphic and structural framework, in: AAPG Studies in Geology 7: Framework, Facies, and Oil-Trapping Characteristics of the Upper Continental Margin, edited by: Coleman, J., Bouma, A. H., and Moore, G., American Association of Petroleum Geologists, Tulsa, OK, 21–42, https://doi.org/10.1306/St7399C2, 1978.
McGuire, J.: Seismic cycles and earthquake predictability on East Pacific Rise transform faults, B. Seismol. Soc. Am., 98, 1067–1084, https://doi.org/10.1785/0120070154, 2008.
McNeill, L. C., Shillington, D. J., Carter, G. D. O., and the Expedition 381 Participants: Corinth Active Rift Development, Proc. IODP, 381, https://doi.org/10.14379/iodp.proc.381.2019, 2019.
Métois, M., Socquet, A., and Vigny, C.: Interseismic coupling, segmentation and mechanical behavior of the central Chile subduction zone, J. Geophys. Res.-Sol. Ea., 117, B03406, https://doi.org/10.1029/2011JB008736, 2012.
Minson, S. E., Brooks, B. A., Glennie, C. L., Murray, J. R., Langbein, J. O., Owen, S. E., Heaton, T. H., Iannucci, R. A., and Hauser, D. L.: Crowdsourced earthquake early warning, Sci. Adv., 1, e1500036, https://doi.org/10.1126/sciadv.1500036, 2015.
Moreno, M., Haberland, C., Oncken, O., Rietbrock, A., Angiboust, S., and Heidbach, O.: Locking of the Chile subduction zone controlled by fluid pressure before the 2010 earthquake, Nat. Geosci., 7, 292–296, https://doi.org/10.1038/ngeo2102, 2014.
Morgan, J., Gulick, S., Mellett, C. L., Green, S. L., and the Expedition 364 Scientists: Chicxulub: Drilling the K-Pg impact crater, Proc. IODP, 364, https://doi.org/10.14379/iodp.proc.364.2017, 2017.
Morris, J. T. and Renken, K. A.: Past, present, and future nuisance flooding on the Charleston peninsula, PLOS One, 15, e0238770, https://doi.org/10.1371/journal.pone.0238770, 2020.
Mountain, G., Proust, J.-N., McInroy, D., Cotterill, C., and the Expedition 313 Scientists: New Jersey Shallow Shelf, Proc. IODP, 313, https://doi.org/10.2204/iodp.proc.313.2010, 2010.
National Geophysical Data Center/World Data Service (NGDC/WDS): NCEI/WDS Global Significant Earthquake Database, NOAA National Centers for Environmental Information [data set], https://doi.org/10.7289/V5TD9V7K, 2023a.
National Geophysical Data Center/World Data Service (NGDC/WDS): NGDC/WDS Global Historical Tsunami Database, NOAA National Centers for Environmental Information [data set], https://doi.org/10.7289/V5PN93H7, 2023b.
National Ocean Service (NOS)/Center for Operational Oceanographic Products & Services (CO-OPS): Tsunami Capable Tide Stations, National Oceanic and Atmospheric Administration (NOAA) [data set], https://tidesandcurrents.noaa.gov/tsunami/ (last access: 29 September 2023), 2023.
Nixon, C. W., McNeill, L. C., Bull, J. M., Bell, R. E., Gawthorpe, R. L., Henstock, T. L., Christodoulou, D., Ford, M., Taylor, B., Sakellariou, D., Ferentinos, G., Papatheodorou, G., Leeder, M. R., Coller, R. E. L. I., Goodliffe, A. M., Sachpazi, M., and Kranis, H.: Rapid spatiotemporal variations in rift structure during development of the Corinth Rift, central Greece, Tectonics, 35, 1225–1248, https://doi.org/10.1002/2015TC004026, 2016.
Özel, N. M., Necmioglu, O., Ergintav, S., Özel, O., Italiano, F., Favali, P., Bigarre, P., Cakir, Z., Geli, L., Aochi, H., Bossu, R., Zulfikar, C., and Sesetyan, K.: MARSite-Marmara Supersite: accomplishments and outlook, EGU General Assembly 2017, 23–28 April 2017, Vienna, Austria, EGU2017-18891, 2017.
Pan, S., Naliboff, J., Bell, R., and Jackson, C.: Bridging spatiotemporal scales of normal fault growth during continental extension using high-resolution 3D numerical models, Geochem. Geophys. Geosyst., 23, e2021GC010316, https://doi.org/10.1029/2021GC010316, 2022.
Popenoe, P., Schmuck, E. A., and Dillon, W. P.: The Cape Fear landslide: slope failure associated with salt diapirism and gas hydrate decomposition, in: Submarine Landslides: Selected Studies in the U.S. Exclusive Economic Zone, edited by: Schwab, W. C., Lee, H. J., and Twichell, D. C., U.S. Geological Survey, Washington, D.C., 40–53, https://doi.org/10.3133/b2002, 1993.
Ruff, L. J.: Do trench sediments affect great earthquake occurrence in subduction zones?, Pure Appl. Geophys., 129, 263–282, https://doi.org/10.1007/BF00874629, 1989.
Ruiz, S., Metois, M., Fuenzalida, A., Ruiz, J., Leyton, F., Grandin, R., Vigny, C., Madariaga, R. and Campos, J.: Intense foreshocks and a slow slip event preceded the 2014 Iquique Mw 8.1 earthquake, Science, 345, 1165–1169, https://doi.org/10.1126/science.1256074, 2014.
Sainsbury, E. M., Schiemann, R. K. H., Hodges, K. I., Shaffrey, L. C., Baker, A. J., and Bhatia, K. T.: How important are post-tropical cyclones for European windstorm risk?, Geophys. Res. Lett., 47, e2020GL089853, https://doi.org/10.1029/2020GL089853, 2020.
Saito, T. and Noda, A.: Mechanically coupled areas on the plate interface in the Nankai Trough, Japan and a possible seismic and aseismic rupture scenario for megathrust earthquakes, J. Geophys. Res.-Sol. Ea., 127, e2022JB023992, https://doi.org/10.1029/2022JB023992, 2022.
Sawyer, D. E. and DeVore, J. R.: Elevated shear strength of sediments on active margins: evidence for seismic strengthening, Geophys. Res. Lett., 42, 10216–10221, https://doi.org/10.1002/2015GL066603, 2015.
Scharroo, R., Smith, W. H. F., and Lillibridge, J. L.: Satellite altimetry and the intensification of Hurricane Katrina, Eos Trans. AGU, 86, 366, https://doi.org/10.1029/2005EO400004, 2005.
Scholz, C. H.: The Mechanics of Earthquakes and Faulting, 3rd edn., Cambridge University Press, Cambridge, UK, https://doi.org/10.1017/9781316681473, 2019.
Schulten, I., Mosher, D. C., Piper, D. J. W., and Krastel, S.: A Massive Slump on the St. Pierre Slope, A New Perspective on the 1929 Grand Banks Submarine Landslide, J. Geophys. Res.-Sol. Ea., 124, 7538–7561, https://doi.org/10.1029/2018JB017066, 2019.
Sparkes, R., Tilmann, F., Hovius, N., and Hillier, J.: Subducted seafloor relief stops rupture in South American great earthquakes: Implications for rupture behaviour in the 2010 Maule, Chile earthquake, Earth Planet. Sci. Lett., 298, 89–94, https://doi.org/10.1016/j.epsl.2010.07.029, 2010.
Stein, S., Geller, R. J., and Liu, M.: Why earthquake hazard maps often fail and what to do about it, Tectonophys, 562–563, 1–25, https://doi.org/10.1016/j.tecto.2012.06.047, 2012.
Taylor, S. K., Bull, J. M., Lamarche, G., and Barnes, P. M.: Normal fault growth and linkage in the Whakatane Graben, New Zealand, during the last 1.3 Myr, J. Geophys. Res., 109, B02408, https://doi.org/10.1029/2003JB002412, 2004.
Telesca, L.: Time-clustering of natural hazards, Nat. Hazards, 40, 593–601, https://doi.org/10.1007/s11069-006-9023-z, 2007.
Urlaub, M., Talling, P. J., and Masson, D. G.: Timing and frequency of large submarine landslides: implications for understanding triggers and future geohazard, Quaternary Sci. Rev., 72, 63–82, https://doi.org/10.1016/j.quascirev.2013.04.020, 2013.
van Rijsingen, E., Lallemand, S., Peyret, M., Arcay, D., Heuret, A., Funiciello, F., and Corbi, F.: How subduction interface roughness influences the occurrence of large interplate earthquakes, Geochem. Geophys. Geosyst., 19, 2342–2370, https://doi.org/10.1029/2018GC007618, 2018.
Vega, A. J., Miller, P. W., Rohli, R. V., and Heavilin, J.: Synoptic climatology of nuisance flooding along the Atlantic and Gulf of Mexico coasts, USA, Nat. Hazards, 105, 1281–1297, https://doi.org/10.1007/s11069-020-04354-5, 2021.
Wallace, L. M., Barnes, P., Beavan, J., Van Dissen, R., Litchfield, N., Mountjoy, J., Langridge, R., Lamarche, G., and Pondard, N.: The kinematics of a transition from subduction to strike-slip: An example from the central New Zealand Plate boundary, J. Geophys. Res.-Sol. Ea., 117, B02405, https://doi.org/10.1029/2011JB008640, 2012.
Wallace, L. M., Saffer, D. M., Barnes, P. M., Pecher, I. A., Petronotis, K. E., LeVay, L. J., and the Expedition 372/375 Scientists: Hikurangi Subduction Margin Coring, Logging, and Observatories, Proc. IODP, 372B/375, https://doi.org/10.14379/iodp.proc.372B375.2019, 2019.
Walsh, K. J. E., McBride, J. L., Klotzbach, P. J., Balachandran, S., Camargo, S. J., Holland, G., Knutson, T. R., Kossin, J. P., Lee, T., Sobel, A., and Sugi, M.: Topical cyclones and climate change, WIREs Climate Change, 7, 65–89, https://doi.org/10.1002/wcc.371, 2016.
Wang, K. and Bilek, S. L.: Do subducting seamounts generate or stop large earthquakes?, Geology, 39, 819–822, https://doi.org/10.1130/G31856.1, 2011.
Webster, J. M., Yokoyama, Y., Cotterill, C., and the Expedition 325 Scientists: Great Barrier Reef Environmental Changes, Proc. IODP, 325, https://doi.org/10.2204/iodp.proc.325.2011, 2011.
Wells, D. L. and Coppersmith, K. J.: New empirical relationships among magnitude, rupture length, rupture width, rupture area, and surface displacement, B. Seismol. Soc. Am., 84, 974–1002, 1994.