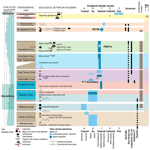
Deep-time Arctic climate archives: high-resolution coring of Svalbard's sedimentary record – SVALCLIME, a workshop report
Denise Kulhanek
Morgan T. Jones
Aleksandra Smyrak-Sikora
Sverre Planke
Valentin Zuchuat
William J. Foster
Sten-Andreas Grundvåg
Henning Lorenz
Micha Ruhl
Kasia K. Sliwinska
Madeleine L. Vickers
We held the MagellanPlus workshop SVALCLIME “Deep-time Arctic climate archives: high-resolution coring of Svalbard's sedimentary record”, from 18 to 21 October 2022 in Longyearbyen, to discuss scientific drilling of the unique high-resolution climate archives of Neoproterozoic to Paleogene age present in the sedimentary record of Svalbard. Svalbard is globally unique in that it facilitates scientific coring across multiple stratigraphic intervals within a relatively small area. The polar location of Svalbard for some of the Mesozoic and the entire Cenozoic makes sites in Svalbard highly complementary to the more easily accessible mid-latitude sites, allowing for investigation of the polar amplification effect over geological time.
The workshop focused on how understanding the geological history of Svalbard can improve our ability to predict future environmental changes, especially at higher latitudes. This topic is highly relevant for the ICDP 2020–2030 Science Plan Theme 4 “Environmental Change” and Theme 1 “Geodynamic Processes”. We concluded that systematic coring of selected Paleozoic, Mesozoic, and Paleogene age sediments in the Arctic should provide important new constraints on deep-time climate change events and the evolution of Earth's hydrosphere–atmosphere–biosphere system.
We developed a scientific plan to address three main objectives through scientific onshore drilling on Svalbard:
- a.
Investigate the coevolution of life and repeated icehouse–greenhouse climate transitions, likely forced by orbital variations, by coring Neoproterozoic and Paleozoic glacial and interglacial intervals in the Cryogenian (“Snowball/Slushball Earth”) and late Carboniferous to early Permian time periods.
- b.
Assess the impact of Mesozoic Large Igneous Province emplacement on rapid climate change and mass extinctions, including the end-Permian mass extinction, the end-Triassic mass extinction, the Jenkyns Event (Toarcian Oceanic Anoxic Event), the Jurassic Volgian Carbon Isotopic Excursion and the Cretaceous Weissert Event and Oceanic Anoxic Event 1a.
- c.
Examine the early Eocene hothouse and subsequent transition to a coolhouse world in the Oligocene by coring Paleogene sediments, including records of the Paleocene–Eocene Thermal Maximum, the Eocene Thermal Maximum 2, and the Eocene–Oligocene transition.
The SVALCLIME science team created plans for a 3-year drilling programme using two platforms: (1) a lightweight coring system for holes of ∼ 100 m length (4–6 sites) and (2) a larger platform that can drill deep holes of up to ∼ 2 km (1–2 sites). In situ wireline log data and fluid samples will be collected in the holes, and core description and sampling will take place at The University Centre in Svalbard (UNIS) in Longyearbyen.
The results from the proposed scientific drilling will be integrated with existing industry and scientific boreholes to establish an almost continuous succession of geological environmental data spanning the Phanerozoic. The results will significantly advance our understanding of how the interplay of internal and external Earth processes are linked with global climate change dynamics, the evolution of life, and mass extinctions.
- Article
(8711 KB) - Full-text XML
- BibTeX
- EndNote
The rate and magnitude of current global warming is unparalleled in the historical record and is forecast to disproportionately affect the polar regions, a process known as “polar amplification” (e.g. Holland and Bitz, 2003). Yet, climate models are known to have difficulty reproducing the latitudinal temperature gradients evidenced by deep-time palaeoclimate studies (e.g. Evans et al., 2018; Price et al., 2020), implying that this polar amplification effect may be even more severe than climate models predict. Detailed knowledge of Earth system dynamics is required to improve these climate models, and the only way to understand both long- and short-term feedback processes is through examination of the geological record of palaeoclimate change. Indeed, the Intergovernmental Panel on Climate Change (IPCC) assessment reports note that palaeoclimate proxy records “extend beyond the variability of recent decadal climate oscillations and thus provide an independent perspective on feedbacks between climate and carbon cycle dynamics” (IPCC, 2021), especially for times of global climate and environmental change. Thus, there is a critical need to study past climate archives in different parts of the globe.
Both the International Continental Scientific Drilling Program (ICDP) and the International Ocean Discovery Program (IODP) science plans highlight the study of past climate archives. Theme 4 (Environmental Change) of the ICDP 2020–2030 Science Plan specifically targets sedimentary archives to understand Earth's evolution and future climate change. In particular, one key question addresses examining past greenhouse conditions to better understand future climate change. The IODP 2050 Science Framework (Koppers and Coggon, 2020) includes the strategic objectives “Earth's Climate System”, “Feedbacks in the Earth System”, and “Tipping Points in Earth's History”, as well as the flagship initiative “Ground truthing future climate change”. Unfortunately with the plan to retire the R/V JOIDES Resolution in 2024, scientific ocean drilling will be limited to mission-specific platform and D/V Chikyu expeditions to address the 2050 Science Framework, making it even more critical to find alternative onshore targets, including Svalbard, to constrain palaeoclimate.
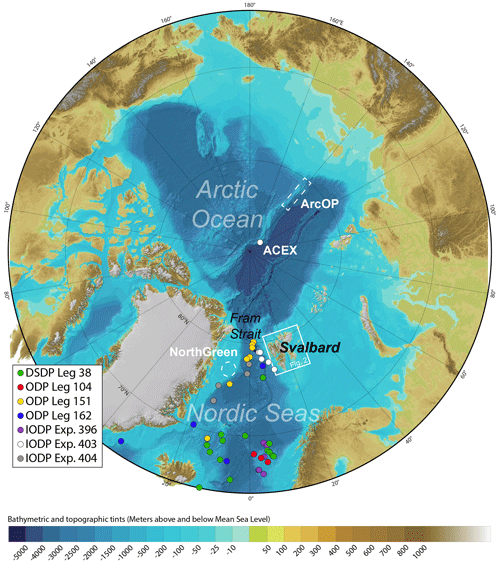
Figure 1Location of Svalbard between the Arctic Ocean and the Nordic Seas and the position of scientific ocean drilling sites in the area. Sites from the most recent IODP expeditions (ACEX, 396, 403, and the cancelled 404) are marked. Potential site positions of the ArcOp and NorthGreen proposals are outlined with dashed white lines. Older DSDP and ODP sites in the Arctic Ocean and Nordic Seas area are indicated with coloured circles. Map from IBCAO (Jakobsson et al., 2012).
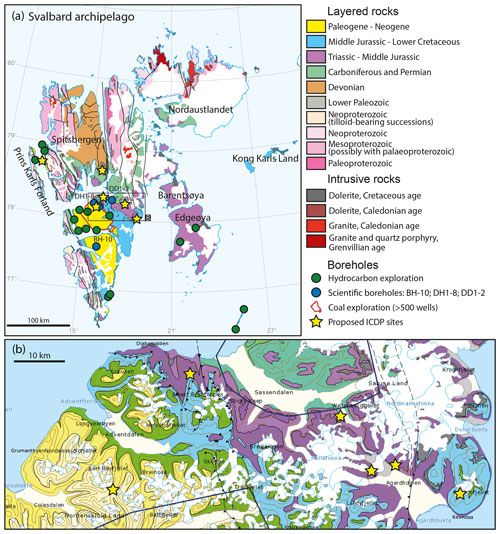
Figure 2(a) Geological map of Svalbard, with locations of previous exploration and scientific wells, and proposed ICDP sites indicated. (b) A local map of central Spitsbergen showing the location of potential ICDP sites (maps from the Norwegian Polar Institute).
The Svalbard archipelago in the Norwegian high Arctic (Figs. 1 and 2) offers a series of unique, continuous, and high-quality sedimentary successions that include numerous global palaeoclimate change events from the last 650 million years (Myr). The outcropping geological successions record both temporally and spatially changing climatic and environmental conditions contemporaneous with Svalbard's drift from the Southern Hemisphere in the Neoproterozoic to its current position at 78∘ N (Fig. 2). Although Svalbard's palaeolatitudinal position varied over time, throughout the Phanerozoic it was located at the northern margin of major landmasses, with a rock record complementary to the more studied successions at lower latitude positions. Because Svalbard drifted to its present position during the Cenozoic, it naturally provides an opportunity to constrain the polar amplification effect in the geological past, e.g. during the Paleocene–Eocene Thermal Maximum (PETM). Evidence of past climatic variations include (i) tillites and carbonates related to the Cryogenian and Ediacaran glacial events; (ii) deposits illustrating the late Paleozoic tropical to subtropical climate transition and eustatic sea-level fluctuations caused by Gondwana glaciations; (iii) the record of the end-Permian mass extinction (EPME); (iv) Mesozoic climate and carbon cycle perturbations including the Dienerian Crisis, Carnian Pluvial Event, end-Triassic mass extinction, Jurassic oceanic anoxic events (OAEs), Weissert Event, Volgian Isotopic Carbon Excursion (VOICE), and OAE1a (Selli Event); (v) the Paleogene succession including rapid climate warming such as the PETM; and (vi) the remains of Quaternary to Holocene glaciations (Fig. 3).
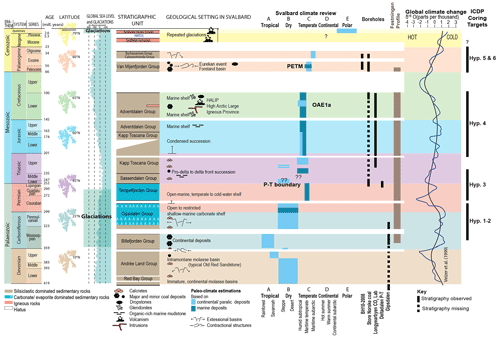
Figure 3Stratigraphic column and climate summary of Svalbard, highlighting the nearly continuous sedimentary record from the Devonian to the Neogene (modified from Smyrak-Sikora et al., 2021). The stratigraphic coverage of research boreholes is indicated, with proposed ICDP drilling targets at right.
Well-exposed outcrops in Svalbard provide excellent targets for analyses of past climate change, and several boreholes have already been drilled and fully cored in Svalbard (Fig. 1). Eight fully cored research boreholes were drilled as part of a CO2 storage project that offer a total of 4.5 km of Triassic–Cretaceous core material (Olaussen et al., 2019), along with supplementary wireline logs. Hundreds of coal exploration boreholes have been drilled mainly within the Paleocene succession with a few providing high-resolution data across the PETM (Dypvik et al., 2011; Senger et al., 2019). Two shallow stratigraphic boreholes from Deltadalen (Zuchuat et al., 2020), with cores of almost 100 m each, allowed for multidisciplinary analyses of the palaeoclimatic conditions across the Permian–Triassic boundary and the accompanying EPME at a resolution not yet undertaken elsewhere in the high Arctic. The Deltadalen research drilling campaign proved that cost- and time-effective stratigraphic drilling and full coring can be achieved with minimal environmental impact. Overall, the core data in combination with nearby outcrop data have formed the basis of numerous spin-off studies, including a re-evaluation of the global stratigraphic timescale. However, the large number of cored boreholes is limited to only a few stratigraphic intervals, have a small diameter (limiting sample material), and are heavily biased by commercial interests and priorities (see Fig. 3 in Senger et al., 2019, for an overview). In addition, many of the existing cores have been poorly stored, making them unusable for palaeoclimate and environmental proxy studies.
To take advantage of existing outcrop and core material and to design future deep-climate research programmes in Svalbard, we held a MagellanPlus ICDP/IODP workshop at The University Centre in Svalbard (UNIS) in Longyearbyen from 18 to 21 October 2022. The main goal of the workshop was to develop new scientific drilling proposals to access Svalbard's unique and well-preserved high Arctic rock record. This overall goal was split into several specific objectives related to the individual deep-time climatic events that require dedicated drilling efforts to acquire relevant drill cores, while others will benefit from pre-existing core material already acquired by the SVALCLIME proponents (Olaussen et al., 2019; Zuchuat et al., 2020).
The workshop (4 workshop days) was attended by 59 in-person participants (from 13 countries) and 33 virtual attendees (from a total of 17 countries), representing diversity in terms of expertise, career stage, gender, and country (see participant list in Appendix A). The workshop was organized with plenary presentations on the first 2 event days, followed by breakout group discussions covering different geological time intervals to identify knowledge gaps and objectives targeting globally significant climate change events. Each represents an objective of global significance that can only be fully achieved through scientific drilling to utilize high-resolution geochemical and non-destructive techniques (e.g. X-ray fluorescence (XRF) core scanning, hyperspectral and computed tomography (CT) imaging, multisensor core logger (MSCL) physical properties) that are not possible with weathered outcrop samples. Further work over the coming year, together with feedback from the ICDP Science Advisory Group (SAG) on a pre-proposal submitted in January 2023, will help to guide which objectives will become the focus of one or more full ICDP drilling proposals. The targets and objectives identified during the MagellanPlus workshop are outlined below by age.
The primary targets for potential future drilling include:
- a.
Cryogenian–Ediacaran tillites and carbonates deposited during glacial or interglacial conditions,
- b.
Carboniferous–Permian transition from coal-rich continental deposits to mixed carbonate–evaporite–siliciclastic rocks that record a shift from a tropical humid climate to an arid subtropical climate,
- c.
Permian–Triassic siliciclastic deposits that record the EPME and its aftermath,
- d.
Jurassic and Cretaceous rocks that record environmental changes associated with igneous intrusions of the High Arctic Large Igneous Province (HALIP; Early Cretaceous) and other LIPs that generated massive quantities of greenhouse gases through contact metamorphism, and
- e.
Paleogene strata that record key climate perturbations such as the PETM and gradual change from hothouse to coolhouse conditions across the Eocene–Oligocene transition (EOT).
While some scientific boreholes under the auspices of DSDP, ODP, and IODP targeted areas near Svalbard (Fig. 1), no ICDP borehole campaign has yet been undertaken on Svalbard. In addition, shallow stratigraphic boreholes were drilled and fully cored off shore in the northern Barents Shelf for petroleum exploration (Lundschien et al., 2024).
Table 1Synthesis of past onshore drilling on Svalbard and its relevance to deep-time palaeoclimate research.
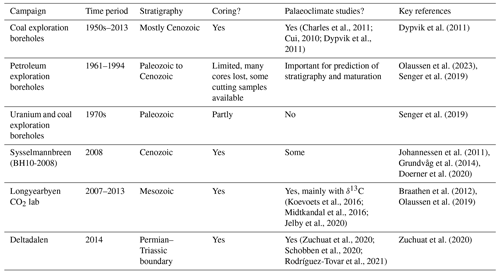
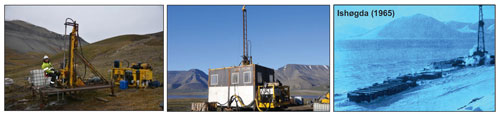
Figure 4Photographs of selected drill sites highlighting the large magnitude of the drilling operations The Permian–Triassic research drilling in Deltadalen was conducted using a helicopter-transportable drill rig and took 1 week to finish. The Longyearbyen CO2 lab boreholes in Adventdalen were drilled and fully cored with coal exploration drill rigs and took about a month to complete. The deepest onshore borehole on Svalbard, Ishøgda, was drilled (but not cored) on the shore of Van Mijenfjorden using a 900 t petroleum exploration drill rig brought in from Canada. Photo credits: Sverre Planke (Deltadalen), Geir Ove Titlestad (Longyearbyen CO2 lab), and Carl A. Wendt (Ishøgda, kindly provided by Svalbard Museum). Refer to Senger et al. (2019) for details on all the boreholes.
Many onshore boreholes have been drilled on Svalbard for coal, petroleum, uranium, and gold exploration as well as scientific research (Table 1; Senger et al., 2019). The scientific boreholes provide drill cores suitable for deep-time palaeoclimate research and were presented during the SVALCLIME workshop. The deep petroleum exploration boreholes provide important constraints on stratigraphic thicknesses, lithology, and maturation and thus form an important foundation for subsequent research drilling. The Deltadalen campaign was undertaken using a helicopter-transportable drilling rig (Fig. 4; Zuchuat et al., 2020) and was completed in 1 week with minimal environmental impact, thus representing a model for some of the proposed sites within the SVALCLIME initiative.
During the workshop, we identified a number of climate events to target through systematic scientific drilling of key stratigraphic intervals on Svalbard. Here we outline these events by age from oldest to youngest, including testable hypotheses and supporting scientific questions developed for the more mature targets.
3.1 Neoproterozoic and Paleozoic
The Cryogenian (720–635 Ma; all ages hereunder are from the International Chronostratigraphic Chart June 2023) spans two of Earth's most extreme glacial intervals, the Sturtian and Marinoan as well as their intervening interglacial (Hoffman and Schrag, 2002; Rooney et al., 2015). Coring this time interval will help to improve age constraints for the Cryogenian using cyclostratigraphy and rhenium-osmium (Re-Os) dating. It would also allow us to further assess the C27–C29 sterane transition (to distinguish biogenic sources of organic matter) that represents a major change in marine primary production from cyanobacteria to eukaryotic algae that occurred during the interglacial (Brocks et al., 2017). There are few cores globally spanning the Cryogenian and none from Svalbard, making this an important target to better understand this period in Earth's history when significant ice existed in tropical regions, as well as how the transition out of “Slushball Earth” impacted the evolution of life in the Ediacaran (635–538.8 Ma). However, the potential drill sites are in remote and largely protected sites; therefore, planning for drilling these targets is not as mature as for the Paleozoic and younger sites.
The Late Paleozoic Ice Age (LPIA) was one of the biggest climatic events of the Phanerozoic as it significantly altered both climate and depositional systems on Earth (Montañez and Poulsen, 2013). It represents the only complete greenhouse–icehouse–greenhouse cycle on a vegetated Earth (cf. Isbell et al., 2008) and is considered a close analogue to present climate conditions with sustained low pCO2 within the range proposed for the near future (Montañez and Poulsen, 2013). The LPIA was characterized by discrete periods of glaciations separated by warm periods (Isbell et al., 2008; Gastaldo et al., 1996; Montañez et al., 2007). The glaciations started during the Visean (346.7–330.9 Ma) and extended into the, most probably, late Permian for more than 100 Myr. During this time interval, Svalbard drifted from a tropical position into the northern subtropical arid zone (Torsvik and Cocks, 2019) and deposition gradually shifted from humid terrestrial to semi-arid terrestrial and finally to shallow marine (Steel and Worsley, 1984). The impact of the glaciations and deglaciation during the LPIA is most readily recognized in the Bashkirian (323.2–315.2 Ma; Pennsylvanian) to Sakamarian (293.52–290.1 Ma; Cisuralian) marine successions of the Gipsdalen Group (Ahlborn and Stemmerik, 2015; Sorento et al., 2020; Smyrak-Sikora et al., 2021; Cutbill and Challinor, 1965). Over 130 cycles of sea-level variations could reveal the magnitude of glacioeustatic sea-level changes and the dynamics of the LPIA, especially the early Permian icehouse–greenhouse transition. Obtaining a fully cored record through these > 130 cycles would contribute to further development of the late Paleozoic timescale and the sensitivity of the planet to external forcings and feedbacks. Thus, we propose to collect ∼ 1000 m of core through this interval to address the following hypotheses and specific scientific questions:
Hypothesis 1. The cycles present in the Gipsdalen Group record orbital cyclicity and its imprint on Earth's climate.
- 1a.
Does the cyclicity relate to a long eccentricity cycle during the Pennsylvanian (Aretz et al., 2020, and references therein), as is inferred for other locations (e.g. China, United States, Ireland)?
- 1b.
How do these cycles correlate with the North American cyclothems (and records from other regions), and what are the implications for the Carboniferous timescale?
Hypothesis 2. The far-field records of LPIA in Svalbard cover the transition across greenhouse–icehouse–peak-icehouse–greenhouse conditions.
- 2a.
Does the gradual climate shift from humid to arid conditions recorded on Svalbard in the Visean represent the environmental and climatic far-field response to the onset of icehouse conditions?
3.2 Mesozoic
The Permian was marked by major supra-regional environmental perturbations including the Artinskian–Kungurian faunal turnover (∼ 283.5 Ma; Lee et al., 2022), the Capitanian mass extinction (∼ 262 Ma; Bond et al., 2020), and EPME (Wignall et al., 1998; Mørk et al., 1999). The subsequent Mesozoic saw enhanced volcanism due to the breakup of Pangea, resulting in major perturbations to the global carbon cycle that led to dramatic and short-lived global warming, significant changes in global hydrological cycling at times, enhanced development of oceanic anoxia especially in marginal marine basins, and extinction events (Schlanger and Jenkyns, 1976; Erba et al., 2015; Herrle et al., 2015; Jelby et al., 2020).
The environments and lithologies in which magmatic emplacement occurs are key factors for atmospheric volatile loading and global climate change. Svensen et al. (2004) showed that emplacement of magma into organic-rich sedimentary basins could create > 10 times the amount of greenhouse gases (CH4 and CO2) than a comparable volume of lava degassing subaerially. For instance, emplacement of magma into the organic- and evaporite-rich Tunguska Basin in Siberia released large volumes of toxic halocarbons through pipe structures called hydrothermal vent complexes, resulting in the largest known Phanerozoic extinction event, the EPME (Svensen et al., 2009). A similar scenario has been proposed for the EPME, whereby massive quantities of toxic and greenhouse gases were released from the Amazon, Parnaíba, and Solimoes basins in South America (Ruhl and Kürschner, 2011; Heimdal et al., 2019).
The Svalbard stratigraphy provides a unique Arctic record to study both the long- and short-term climate variations associated with such global perturbations in the Mesozoic. Indeed, most of what is known about the EPME is based on records from China, southern Europe, and the Middle East, which all represent subtropical, Tethyan successions (e.g. China and central Europe; Jin et al., 2000; Farabegoli et al., 2007). Yet, the fossil record and modelling studies suggest that the impact of the climate crisis was greater at higher latitudes and along the Panthalassan margin of Pangea (Penn et al., 2018). During the late Permian and Triassic, Svalbard was located on the northern margin of Pangea between ∼ 45 and 50∘ N (Elvevold et al., 2007). On Svalbard, the Permian strata of the Kapp Starostin Formation (Cutbill and Challinor, 1965) was deposited in a cold-water ramp setting (Bond et al., 2018). The conformably overlying Triassic succession consists of nine high-sedimentation-rate, fining-up transgressive, and coarsening-up regressive cycles (Mørk et al., 1989; Vigran et al., 2014) associated with progradational pulses of the “largest delta … in Earth's history” across the Barents Shelf (Klausen et al., 2019). Svalbard is unique compared to the equatorial, Tethyan successions in that it records diverse marine ecosystems but also silicified fauna (Foster et al., 2017) and faunal groups currently unknown from coeval shallow-marine settings, including radiolarians (Foster et al., 2023), hexactinellid sponges (Foster et al., 2023), red algae (Wignall et al., 1998), and bryozoans (Nakrem and Mørk, 1991). The absence of these groups from intensely studied equatorial shallow-marine successions from the Tethys and Panthalassa oceans suggests that their absence in these equatorial settings represents a biological signal and more habitable conditions in the Boreal Realm that can only be studied in difficult-to-access locations, including Arctic Canada, Greenland, and Russia.
The Permian–Triassic boundary succession was cored in two boreholes at Deltadalen (DD1-2; Fig. 2a; ∼ 90 m each), providing a good record of the mass extinction event and its aftermath. However, the glauconitic, chert-rich, and often thoroughly bioturbated sandstone facies of the upper Permian Kapp Starostin Formation prevented detailed palynological, geochemical, and palaeoenvironmental analyses of pre-EPME conditions (Zuchuat et al., 2020). In addition, because of the high sedimentation rates, the 90 m core only covers the entire Induan (251.902–251.2 Ma) and the lower part of the Olenekian stages of the Lower Triassic. Therefore, most of the climatic events of the Triassic (including the Dienerian Crisis (upper Induan) or the Carnian Pluvial Event ∼ 234 Ma; Ogg et al., 2016) are yet to be investigated (Fig. 2). We therefore propose to core a ∼ 700 m succession spanning from the Upper Triassic strata into the Permian Kapp Starostin Formation from the top of and at the foot of Kropotkinfjellet, Friedrichfjellet, or Roslagenfjellet in Agardhdalen (Fig. 2b) to address the following hypothesis and key questions:
Hypothesis 3. The Svalbard sedimentary sequences record environmental changes from the Permian through Late Triassic associated with global perturbations in the carbon cycle resulting from major tectonic events, including the eruption and emplacement of the Siberian Traps Large Igneous Province (LIP) and the breakup of Pangea.
- 3a.
How is the EPME expressed at higher latitudes relative to the equatorial regions?
- 3b.
Are subsequent Triassic environmental perturbations and associated carbon isotope excursions (CIEs) present in low-latitude sections also found in Svalbard, suggesting a global nature?
- 3c.
How do the progradational pulses of the delta migrating over the Barents Shelf recorded in the Triassic strata of Svalbard tie to concomitant global environmental perturbations, such as changes in regional or global hydrological cycling?
Mechanisms for the formation of Jurassic and Cretaceous OAEs are still debated, but the synchronicity between LIP emplacement and these events implies either a direct causal link (e.g. similar to the scenario above) or that greenhouse gas emissions from these LIPs drove global temperatures beyond a tipping point, and massive methane release from continental shelves drove extreme global climate change and ocean stratification (e.g. Hesselbo et al., 2000). Recent work from outcrops on Svalbard suggest that the subaerial HALIP volcanism may have played a more important role for at least the Northern Hemisphere changes than the larger, but submarine, Greater Ontong-Java Plateau LIP in the Pacific (e.g. Percival et al., 2021; Vickers et al., 2023). Furthermore, a heterogeneously distributed Upper Jurassic CIE (the VOICE event) was recently identified in Arctic regions and corroborated with one South American site; the causes and effects of this event are poorly understood (Jelby et al., 2020; Weger et al., 2022). We propose to core Triassic, Jurassic, and Cretaceous strata suitable for high-resolution proxy studies in key localities near the east coast of Spitsbergen where the thermal effects of the Paleogene fold-and-thrust belt are less severe than in the west to address the following hypothesis and specific questions:
Hypothesis 4. LIPs played a major role in global climate change and biotic crises during the Jurassic and Early Cretaceous.
- 4a.
What are the Arctic climate responses to the Karoo LIP (∼ 182 Ma) and the central Atlantic LIP (∼ 201 Ma)?
- 4b.
What are the mechanisms and drivers of the VOICE and associated Weissert Event in the Valanginian (∼ 133 Ma)?
- 4c.
What is the impact of HALIP intrusions on global carbon cycling and climate during OAE1a (∼ 120 Ma)?
3.3 Paleogene
The Paleogene is a key interval for understanding the role of boreal environments in a changing global climate. This time interval is marked by fluctuations between warm-house and hothouse conditions from the Paleocene to mid-Eocene, followed by gradual cooling that culminated in the establishment of a coolhouse climate by the early Oligocene (Westerhold et al., 2020). The hothouse conditions included periods of transient hyperthermal events such as the PETM at ∼ 56 Ma (Cramer et al., 2003). There is evidence of polar amplification for periods of extreme warmth, with temperate to subtropical flora identified in both marine (Sluijs et al., 2020) and terrestrial archives in the Arctic (Suan et al., 2017; Weijers et al., 2007; West et al., 2015; Willard et al., 2019). Despite the importance of polar regions in understanding global climate evolution through the Paleogene, the marine palaeoclimate record for the Arctic is severely underrepresented in global datasets. Integrated Ocean Drilling Program Exp. 302 (Arctic Coring Expedition, ACEX) to the Lomonosov Ridge (Backman et al., 2004) is currently the only scientific ocean drilling conducted in the Arctic Ocean to date. ACEX sites provide key marine archives for the Paleocene and early Eocene (Sluijs et al., 2020), but there is a considerable hiatus of ∼ 26 Myr in ACEX cores (∼ 44–18 Ma) that extends onto the Barents Shelf (Backman et al., 2008; Sangiorgi et al., 2008). An alternative age model by Poirier and Hillaire-Marcel (2011) suggests that the “hiatus” succession is heavily condensed. Subsequent efforts to redrill in the High Arctic (International Ocean Discovery Program (IODP) Expeditions 377 and 404) have recently been cancelled. As such, there is a major palaeoclimate data gap in this region, with no current drilling opportunities planned in the coming decade. The Svalbard archipelago is an ideal locality to address this knowledge gap. It has been located within the Arctic Circle during the last 60 Myr and contains an expanded and well-preserved succession of shallow marine to deltaic sediments of Paleocene (< 62 Ma) to Oligocene (∼ 25 Ma) age (Helland-Hansen and Grundvåg, 2021). Continuous coring in strategic locations will allow us to test several critical hypotheses and pivotal research questions in Paleogene climate research.
The PETM is marked in the geological record by a large CIE (δ13C), indicative of large atmospheric fluxes of CO2 that caused 4–6 ∘C of global warming (e.g. Inglis et al., 2020; Zachos et al., 2008). The sources of carbon required to instigate the PETM are intensely debated (e.g. Dickens et al., 1995; Lourens et al., 2005; Svensen et al., 2004; Gutjahr et al., 2017). One potential candidate was the emplacement of the North Atlantic Igneous Province (NAIP). There is good temporal agreement between peak NAIP activity and the PETM, and both volcanic and contact metamorphic degassing are large potential carbon sources (Svensen et al., 2004; Jones et al., 2016). However, the exact relationship between the NAIP and the PETM is complicated by a lack of expanded successions that contain both volcanic and climatic proxies. The Central Spitsbergen Basin succession outcropping is an ideal candidate for resolving some of these uncertainties, as the ∼ 2.5 km thick sequence represents near-continuous deposition during the formation of a foreland basin. The relative proximity of Svalbard to the NAIP resulted in the co-preservation of multiple volcanic and climatic proxies. The PETM is within an expanded record of homogeneous siliciclastic mudstone deposited in a shallow to moderately deep marine setting (Dypvik et al., 2011). The lithological homogeneity and lack of extreme variations in sedimentation rates make the Svalbard strata an invaluable locality, as the vast majority of global PETM sites are affected by these factors. The relatively consistent sedimentation rates, high-latitude setting, and presence of some tephra layers mean that a robust geochronology can be attained by cyclostratigraphy, magnetostratigraphy, and radiometric dating. To date, only a single borehole (09/2005) has been studied in detail from the Central Spitsbergen Basin (e.g. Dypvik et al., 2011; Charles et al., 2011; Harding et al., 2011; Cui et al., 2021). Coring a new PETM interval at a location southeast of Longyearbyen (Fig. 2b) will allow for the implementation of state-of-the-art techniques, such as downhole imaging and logging that were unavailable for studies on core 09/2005, and also provides a detailed record of the cessation of elevated magmatic activity into the early Eocene. This new drill core will allow us to address the following hypothesis and key questions:
Hypothesis 5. NAIP activity was a potential driver of rapid and severe global climate change.
- 5a.
Can a high-resolution record from Svalbard improve our understanding of what caused the initiation and long duration of the PETM hyperthermal?
- 5b.
Can refined geochronology distinguish between various carbon sources, such as NAIP eruptions and thermogenic degassing from intrusions, during the evolution of the PETM?
The primary driver of global cooling beginning in the late Eocene is uncertain, with declining atmospheric CO2 and opening of tectonic gateways being the leading hypotheses (e.g. Hutchinson et al., 2021). Unlike the opening of the Tasman Gateway and Drake Passage (e.g. Toggweiler and Bjornsson, 2000; Stickley et al., 2004), there are significant uncertainties in the timing and nature of the opening of the Fram Strait (e.g. von Appen et al., 2015; Hossain et al., 2021). Recent studies suggest that the Eocene–Oligocene transition from warm-house to coolhouse conditions (∼ 34 Ma) may have been controlled by declining atmospheric CO2 concentration, with changing bathymetry of the northern gateways playing a secondary role (e.g. Hutchinson et al., 2019; Straume et al., 2022). The onset of glaciation on Antarctica at ∼ 34 Ma marked the beginning of the icehouse world at one pole. However, the onset of glaciation in the northern high latitudes is uncertain, with some studies arguing for the presence of Arctic sea ice from as early as the mid-Eocene (Stickley et al., 2009; Tripati and Darby, 2018). The lack of marine sedimentary archives from the high Arctic region due to the ∼ 26 Myr data gap in the ACEX core precludes further testing of this hypothesis, along with a host of palaeoenvironmental data that would place Arctic climate evolution in a global context. Therefore, the recent cancellation of IODP Expedition 404 due to the planned decommissioning of the R/V JOIDES Resolution in 2024 dashed the hopes of the scientific community to acquire much needed data from the high Arctic; therefore, alternative targets must be sought.
The Eocene to Oligocene Forlandsundet Graben is a key locality for addressing this knowledge gap. Oblique extension and normal faulting on the western Svalbard margin formed a series of N–S trending basins, of which only the Forlandsundet Graben is, in part, subaerially exposed (Steel et al., 1985; Blinova et al., 2009; Kristoffersen et al., 2020; Schaaf et al., 2021). The activation of faulting and extension may represent a precursor of the Fram Strait and is therefore a key area to unravel the timing and sequence of events that established the seaway. The thickness of the Forlandsundet Graben infill is poorly constrained due to the tectonic complexity of the area and scattered outcrop sections but exceeds 4 km in places (Dallmann, 1999). Age estimates are also uncertain but ages as young as middle-to-late Oligocene have been proposed (Schaaf et al., 2021; Feyling-Hanssen and Ulleberg, 1984). An exploration well drilled near the centre of the graben penetrated > 1 km of mostly marine mudstones and sandstones of Eocene and Oligocene age before reaching metamorphic basement (Senger et al., 2019; Schaaf et al., 2021). Unfortunately, only ∼ 20 m of the entire borehole were cored. Nevertheless, this borehole acts as a proof of concept that a continuous core of the Forlandsundet strata can be obtained to address the following hypothesis and key questions:
Hypothesis 6. The opening of the Fram Strait played a major role in global thermohaline circulation and the cooling of Earth's climate.
- 6a.
Can the use of palaeoceanographic tracers in the Forlandsundet Graben establish when the deep-water connection from the Arctic to global oceans was initiated?
- 6b.
Can drilling in the Forlandsundet Graben provide the first ever Eocene-to-Oligocene marine palaeoclimate archive in the high Arctic region?
Svalbard is the optimal logistical location for coring the Phanerozoic stratigraphy of the Arctic. Longyearbyen is a well-developed and accessible settlement (population 2400) with a university centre (UNIS), tourism infrastructure (e.g. hotels, boats, snowmobiles), and a natural polar logistics centre. Daily scheduled flights connect Longyearbyen to Norway (Oslo and Tromsø), and a mostly ice-free harbour serves weekly transport vessels arriving from the mainland. The archipelago has a more than 100-year-long record of year-round geological exploration and drilling with relatively limited impact on the environment.
Svalbard's legal status is governed by the Svalbard Treaty signed in 1920 and ratified in 1925. The Svalbard Treaty grants Norway full sovereignty over Svalbard, but it also prohibits discrimination against companies from outside Norway accessing Svalbard's natural resources. This is particularly reflected in the diverse international group of companies involved in petroleum exploration in Svalbard from the 1960s to the early 1990s (Senger et al., 2019).
However, environmental regulations have since become much stricter, with large-scale environmental protection implemented in 1973 (establishment of major natural parks and reserves in eastern Svalbard and the full protection of polar bears) and 2001 (through the passing of the Svalbard Environmental Protection Act), constraining the type of activities that are possible and their location. In the context of research drilling, coal exploration drilling around Nordenskiöld Land, deep drilling near Longyearbyen, and shallow drilling at Deltadalen in 2015 all illustrate the feasibility of acquiring high-quality cores with minimal environmental effects.
4.1 Existing data and site selection
An extensive geophysical and geological database exists for Svalbard, called Svalbox (Senger et al., 2022; http://www.svalbox.no/map, last access: 4 October 2023; Betlem et al., 2023; Fig. 5). Data available for SVALCLIME include (a) nearshore marine reflection seismic lines, covering almost all fjords, much of Spitsbergen's shoreline, and parts of the adjacent shelf; (b) onshore seismic reflection lines at key localities, such as Adventdalen, where they extend the offshore seismic lines to image the succession of the Central Spitsbergen Basin; (c) hydrocarbon exploration wells at a number of sites throughout Svalbard, between 200 m and > 3 km deep, mostly uncored; (d) ore exploration boreholes, mainly short intervals cored for coal and concentrated on relevant stratigraphic intervals; and (e) Longyearbyen CO2 lab and Deltadalen drill cores and data (important for operational planning, including lessons learnt from drilling through permafrost and pressurized sections). The SVALCLIME coring targets are primarily stratigraphy that is (1) poorly or not exposed or is highly weathered where exposed, (2) located away from outcrop localities, (3) less altered due to intrusive activity, and (4) is not already fully cored in an optimal location (considering thermal maturity, lithologies, sedimentation rate, basinal position, etc.).
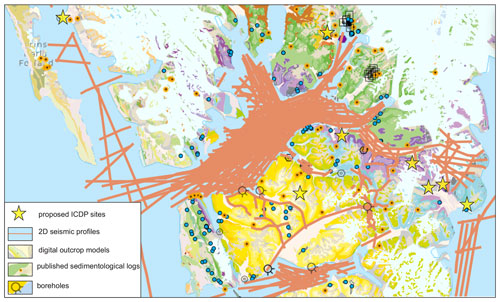
Figure 5Database of existing geoscientific material including existing boreholes, seismic data, and high-resolution digital outcrop models as integrated in the Svalbox platform (Betlem et al., 2023; Senger et al., 2022). The interactive map is accessible at http://www.svalbox.no/map.
4.2 Drilling platforms
During the workshop, we discussed drilling platforms and their suitability for the proposed SVALCLIME drilling targets, which can be divided into two groups: shallow, with a target depth of up to a couple of hundred metres, and deep. Deep targets include the Forlandsundet Graben at Sarstangen with > 1 km target depth (Hypothesis 6) and the Central Spitsbergen Basin near Longyearbyen with > 2 km target depth (Hypothesis 5); a third deep target on the southeastern shore of Sassenfjorden (∼ 1.5 km; Hypothesis 1) can possibly be covered by multiple shallow holes. All other targets belong to the shallow group. Suitable drilling platforms are capable of continuous core drilling to target depth in at least N size (47.6 mm core diameter, 75.7 mm hole diameter with NQ drill bit) and sufficiently adaptable such that all required components can be mobilized to drill sites in comparatively remote Arctic environment without significant negative impact on the local environment. Certain sites for shallow boreholes are located on mountaintops and slopes, which requires transport by helicopter. We identified several potential drilling rigs that meet these requirements: (1) The University Centre in Svalbard (UNIS) operates a drill rig that can be used for short, uncomplicated coring down to 130 m. (2) SNSK (Store Norske, formerly Store Norske Spitsbergen Kulkompani AS, the Norwegian coal and service company on Svalbard) has used a drill rig operated by Arctic Drilling Company Oy Ltd for exploration locations that are only accessible by helicopter. The successor of this rig is expected to have a depth capacity of 200–300 m. (3) Other lightweight platforms for drilling to ∼ 500 m (NQ: drill bit size; gives 47.6 mm core diameter) exist (e.g. underground exploration drill rigs) and could possibly be adapted to the requirements. (4) The Swedish National Research Infrastructure for Scientific Drilling “Riksriggen” can be used for deep core drilling in complicated, challenging environments. This platform is well known from previous ICDP projects (Eger Rift; Collisional Orogeny in the Scandinavian Caledonides (COSC); Lorenz et al., 2022; Fischer et al., 2022) and has a maximum drilling depth of 2.5 km (NQ). Riksriggen is comparatively large and heavy but still easily mobilized when compared to conventional rotary drilling equipment.
4.3 Operational and science planning and safety considerations
SVALCLIME operational planning is being done in collaboration with Riksriggen and SNSK, which became involved in the project during the MagellanPlus workshop. Major safety concerns, in addition to those expected in sedimentary basins, are linked to permafrost and the highly sensitive Arctic environment. Potential dangers, in particular during deep drilling, are fluid and gas overpressure below permafrost and at certain stratigraphic levels, semi-consolidated sediments and swelling clays in certain stratigraphic intervals, and the complications of drilling through multiple fault and décollement zones related to the West Spitsbergen Fold and Thrust Belt. Gas is also present regionally as shale gas in shale-dominated successions such as the Agardhfjellet and Botneheia formations, and this is well characterized in Adventdalen (Ohm et al., 2019). Much can be learnt from the analysis of the drilling reports from previous drilling projects, e.g. hydrocarbon exploration drilling and the Longyearbyen CO2 lab.
Table 2Summary of thematically or geographically complementary scientific projects with relevance to SVALCLIME.
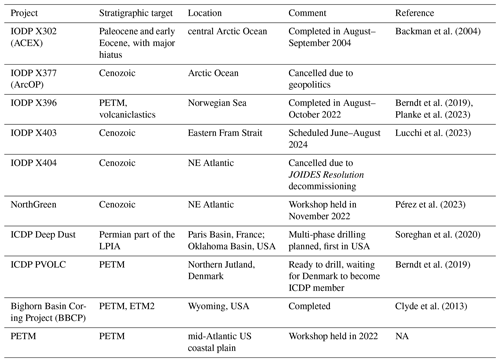
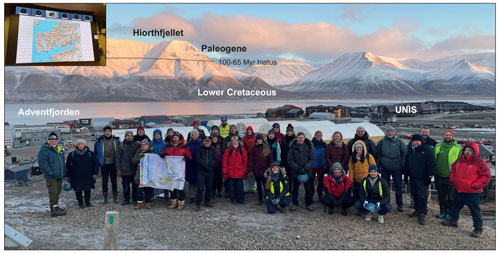
Figure 6Group photograph of the SVALCLIME participants taken during an optional extended lunch break while walking from UNIS to outcrops exposing the Lower Cretaceous Carolinefjellet Formation on the road connecting Longyearbyen with Svalbard Airport.
In our ICDP pre-proposal submitted in January 2023, we proposed a 3-year drilling campaign using two different platforms. In the first year, shallow boreholes (∼ 100 m) will be drilled with a lightweight drilling platform near Longyearbyen. With a similar approach but slightly deeper targets, four sites in more distal locations towards the east coast of Svalbard will be drilled during the second year. Finally, a large rig will be used at 1–2 sites during the third year, with expected drilling depths of between 1 km and more than 2 km. Final scheduling will depend on permitting, logistic, and economic considerations. On-site science will most likely be limited to producing knowledge required for managing the drilling and tasks that cannot be delayed. Scientific work on the drill core, such as high-resolution lithological descriptions, core scanning and logging, and initial sampling and analyses, will be conducted at existing facilities in Longyearbyen. The downhole logging, testing, and sampling plan will ensure that the boreholes will be documented during the drilling phase to secure a maximum of information in case of an unexpected loss of the borehole and that a full scientific coverage to total depth is achieved with post-drilling surveys.
Of paramount importance is timely and sustained contact with the office of the governor of Svalbard, to inform them about the project, discuss targets, and get advice about regulations and restrictions, thus preparing for the permitting process and outreach to the local community.
The SVALCLIME initiative is ambitious in its multi-event approach while geographically constrained to Svalbard itself. As such, it is important to highlight how this broad approach is linked and complementary to other ongoing initiatives on deep-time climate in the region and globally (Table 2).
The outlined SVALCLIME workshop (Fig. 6) fulfilled its main objective by bringing together a broad, multidisciplinary, enthusiastic group of researchers spanning from MSc students to senior scientists and by formulating specific research questions that Svalbard's rock record can contribute to answering. An ICDP pre-proposal was submitted in January 2023 as a direct result of the workshop. A full ICDP drilling proposal will be developed during 2023 and submitted in January 2024, incorporating comments from ICDP's Scientific Advisory Group on the pre-proposal and through further engagement of the international geoscientific community. In addition, we foresee systematic use of existing drill cores in research projects and in relevant courses at UNIS and other involved institutions to complement work on cores obtained through future ICDP drilling.
ACEX | Arctic Coring Expedition (Integrated Ocean Drilling Program Exp. 302) |
ArcOP | Arctic Ocean Paleoceanography |
BBCP | Bighorn Basin Coring Project |
CIE | carbon isotope excursion |
COSC | Collisional Orogeny in the Scandinavian Caledonides |
CT | computed tomography |
DSDP | Deep Sea Drilling Project |
EOT | Eocene–Oligocene transition |
EPME | end-Permian mass extinction |
HALIP | High Arctic Large Igneous Province |
ICDP | International Continental Scientific Drilling Program |
IODP | International Ocean Discovery Program |
IPCC | Intergovernmental Panel on Climate Change |
LIP | large igneous province |
LPIA | Late Paleozoic Ice Age |
MSCL | multisensor core logger |
NAIP | North Atlantic Igneous Province |
OAE | oceanic anoxic event |
ODP | Ocean Drilling Program |
PETM | Paleocene–Eocene Thermal Maximum |
PVOLC | volcanic forcing and Paleogene climate change |
Re-Os | rhenium–osmium |
SAG | Science Advisory Group |
SNSK | Store Norske (formerly Store Norske Spitsbergen Kulkompani AS, the Norwegian coal mining company on Svalbard) |
UNIS | The University Centre in Svalbard |
VOICE | Volgian isotopic carbon excursion |
XRF | X-ray fluorescence |
No data sets were used in this article.
KS, ASS, MTJ, SP, VZ, and DK proposed and convened the workshop. All co-authors contributed to the text and figures that resulted in the submission of a preliminary ICDP proposal, led by DK. Participants at the workshop contributed intellectual input. All co-authors contributed to writing and reviewing the text of this article.
The contact author has declared that none of the authors has any competing interests.
Publisher’s note: Copernicus Publications remains neutral with regard to jurisdictional claims made in the text, published maps, institutional affiliations, or any other geographical representation in this paper. While Copernicus Publications makes every effort to include appropriate place names, the final responsibility lies with the authors.
We sincerely appreciate the participants of the SVALCLIME workshop for their scientific expertise and enthusiasm to develop the SVALCLIME ICDP project. We also thank the UNIS student assistants Tereza Mosočiová, Nil Rodes, Carl Lie, and Matthijs Nuus for support during the workshop, for allowing digital participation, for organizing field excursions, and for the all-important catering.
Finally, we sincerely thank journal reviewers Matt O'Regan, Catalina Gebhardt, and Julie Brigham-Grette for their constructive feedback.
This research has been supported by the Norges Forskningsråd (grant no. 331679). The SVALCLIME workshop was funded by MagellanPlus/ECORD and UNIS. The efforts built largely on the “Svalbard Rock Vault” and “Svalbox” projects, both funded by Svalbard Strategic Grants from the Research Council of Norway.
This paper was edited by Nadine Hallmann and reviewed by Matt O'Regan, Catalina Gebhardt, and Julie Brigham-Grette.
Ahlborn, M. and Stemmerik, L.: Depositional evolution of the Upper Carboniferous–Lower Permian Wordiekammen carbonate platform, Nordfjorden High, central Spitsbergen, Arctic Norway, Norw. J. Geol., 95, 91–126, https://doi.org/10.17850/njg95-1-03, 2015.
Aretz, M., Herbig, H.-G., Wang, X., Gradstein, F., Agterberg, F., and Ogg, J.: The carboniferous period, in: Geologic time scale 2020, Elsevier, 811–874, https://doi.org/10.1016/B978-0-12-824360-2.00023-1, 2020.
Backman, J., Jakobsson, M., Løvlie, R., Polyak, L., and Febo, L. A.: Is the central Arctic Ocean a sediment starved basin?, Quaternary Sci. Rev., 23, 1435–1454, https://doi.org/10.1029/2007PA001476, 2004.
Backman, J., Jakobsson, M., Frank, M., Sangiorgi, F., Brinkhuis, H., Stickley, C., O'Regan, M., Løvlie, R., Pälike, H., Spofforth, D., Gattacecca, J., Moran, K., King, J., and Heil, C.: Age model and core-seismic integration for the Cenozoic Arctic Coring Expedition sediments from the Lomonosov Ridge, Paleoceanography, 23, PA1S03, https://doi.org/10.1029/2007PA001476, 2008.
Berndt, C., Planke, S., Teagle, D., Huismans, R., Torsvik, T., Frieling, J., Jones, M. T., Jerram, D. A., Tegner, C., Faleide, J. I., Coxall, H., and Hong, W.-L.: Northeast Atlantic breakup volcanism and consequences for Paleogene climate change – MagellanPlus Workshop report, Sci. Dril., 26, 69–85, https://doi.org/10.5194/sd-26-69-2019, 2019.
Betlem, P., Rodes, N., Birchall, T., Dahlin, A., Smyrak-Sikora, A., and Senger, K.: The Svalbox Digital Model Database: a geoscientific window to the High Arctic, Geosphere, https://doi.org/10.1130/GES02606.1, online first, 2023.
Blinova, N. V., Stejskal, J., Trchová, M., Sapurina, I., and Ćirić-Marjanović, G.: The oxidation of aniline with silver nitrate to polyaniline–silver composites, Polymer, 50, 50–56, https://doi.org/10.1016/j.polymer.2008.10.040, 2009.
Bond, D. P., Blomeier, D. P., Dustira, A. M., Wignall, P. B., Collins, D., Goode, T., Groen, R. D., Buggisch, W., and Grasby, S. E.: Sequence stratigraphy, basin morphology and sea-level history for the Permian Kapp Starostin Formation of Svalbard, Norway, Geol. Mag., 155, 1023–1039, https://doi.org/10.1017/S0016756816001126, 2018.
Bond, D. P., Wignall, P. B., and Grasby, S. E.: The Capitanian (Guadalupian, Middle Permian) mass extinction in NW Pangea (Borup Fiord, Arctic Canada): A global crisis driven by volcanism and anoxia, GSA Bull., 132, 931–942, https://doi.org/10.1130/B35281.1, 2020.
Braathen, A., Bælum, K., Christiansen, H. H., Dahl, T., Eiken, O., Elvebakk, H., Hansen, F., Hanssen, T. H., Jochmann, M., Johansen, T. A., Johnsen, H., Larsen, L., Lie, T., Mertes, J., Mørk, A., Mørk, M. B., Nemec, W. J., Olaussen, S., Oye, V., Rød, K., Titlestad, G. O., Tveranger, J., and Vagle, K.: Longyearbyen CO2 lab of Svalbard, Norway – first assessment of the sedimentary succession for CO2 storage, Norw. J. Geol., 92, 353–376, 2012.
Brocks, J. J., Jarrett, A. J., Sirantoine, E., Hallmann, C., Hoshino, Y., and Liyanage, T.: The rise of algae in Cryogenian oceans and the emergence of animals, Nature, 548, 578–581, https://doi.org/10.1038/nature23457, 2017.
Intergovernmental Panel on Climate Change (IPCC): Global Carbon and Other Biogeochemical Cycles and Feedbacks, in: Climate Change 2021 – The Physical Science Basis: Working Group I Contribution to the Sixth Assessment Report of the Intergovernmental Panel on Climate Change, Cambridge, Cambridge University Press, 673–816, https://doi.org/10.1017/9781009157896.007, 2023.
Charles, A. J., Condon, D. J., Harding, I. C., Pälike, H., Marshall, J. E., Cui, Y., Kump, L., and Croudace, I. W.: Constraints on the numerical age of the Paleocene-Eocene boundary, Geochem. Geophy. Geosy., 12, Q0AA17, https://doi.org/10.1029/2010GC003426, 2011.
Clyde, W. C., Gingerich, P. D., Wing, S. L., Röhl, U., Westerhold, T., Bowen, G., Johnson, K., Baczynski, A. A., Diefendorf, A., McInerney, F., Schnurrenberger, D., Noren, A., Brady, K., and the BBCP Science Team: Bighorn Basin Coring Project (BBCP): a continental perspective on early Paleogene hyperthermals, Sci. Dril., 16, 21–31, https://doi.org/10.5194/sd-16-21-2013, 2013.
Cramer, B. S., Wright, J. D., Kent, D. V., and Aubry, M. P.: Orbital climate forcing of δ13C excursions in the late Paleocene–early Eocene (chrons C24n–C25n), Paleoceanography, 18, 1097, https://doi.org/10.1029/2003PA000909, 2003.
Cui, Y.: Carbon addition during the Paleocene-Eocene Thermal Maximum: model inversion of a new, high-resolution carbon isotope record from Svalbard, MSc thesis, Pennsylvania State University, https://etda.libraries.psu.edu/files/final_submissions/6907 (last access: 4 October 2023), 2010.
Cui, Y., Diefendorf, A. F., Kump, L. R., Jiang, S., and Freeman, K. H.: Synchronous marine and terrestrial carbon cycle perturbation in the high arctic during the PETM, Paleoceanography and Paleoclimatology, 36, e2020PA003942, https://doi.org/10.1029/2020PA003942, 2021.
Cutbill, J. and Challinor, A.: Revision of the stratigraphical scheme for the Carboniferous and Permian rocks of Spitsbergen and Bjørnøya, Geol. Mag., 102, 418–439, 1965.
Dallmann, W. K. (Ed.): Lithostratigraphic Lexicon of Svalbard. Upper Palaeozoic to Quaternary Bedrock, Review and Recommendations for Nomenclature Use, Committee on the Stratigraphy of Svalbard/Norsk Polarinstitutt, p. 320, ISBN 82-7666-166-1, 1999.
Dickens, G. R., O'Neil, J. R., Rea, D. K., and Owen, R. M.: Dissociation of oceanic methane hydrate as a cause of the carbon isotope excursion at the end of the Paleocene, Paleoceanography, 10, 965–971, 1995.
Doerner, M., Berner, U., Erdmann, M., and Barth, T.: Geochemical characterization of the depositional environment of Paleocene and Eocene sediments of the Tertiary Central Basin of Svalbard, Chem. Geol., 542, 119587, https://doi.org/10.1016/j.chemgeo.2020.119587, 2020.
Dypvik, H., Riber, L., Burca, F., Rüther, D., Jargvoll, D., Nagy, J., and Jochmann, M.: The Paleocene–Eocene thermal maximum (PETM) in Svalbard – clay mineral and geochemical signals, Palaeogeogr. Palaeocl., 302, 156–169, 2011.
Elvevold, S., Dallmann, W., and Blomeier, D.: Geology of Svalbard, Norwegian Polar Institute, Tromsø, Norway, 38 pp., https://brage.npolar.no/npolar-xmlui/bitstream/handle/11250/173141/GeologyOfSvalbard.pdf?sequence=1&isAllowed=y (last access: 4 October 2023), 2007.
Erba, E., Duncan, R. A., Bottini, C., Tiraboschi, D., Weissert, H., Jenkyns, H. C., and Malinverno, A.: Environmental consequences of Ontong Java Plateau and Kerguelen plateau volcanism, The origin, evolution, and environmental impact of oceanic large igneous provinces, Geol. Soc. Am. Spec. Pap., 511, 271–303, https://doi.org/10.1130/2015.2511(15), 2015.
Evans, D., Sagoo, N., Renema, W., Cotton, L. J., Müller, W., Todd, J. A., Saraswati, P. K., Stassen, P., Ziegler, M., and Pearson, P. N.: Eocene greenhouse climate revealed by coupled clumped isotope-Mg/Ca thermometry, P. Natl. Acad. Sci. USA, 115, 1174–1179, https://doi.org/10.1073/pnas.1714744115, 2018.
Farabegoli, E., Perri, M. C., and Posenato, R.: Environmental and biotic changes across the Permian–Triassic boundary in western Tethys: the Bulla parastratotype, Italy, Global Planet. Change, 55, 109–135, https://doi.org/10.1016/j.gloplacha.2006.06.009, 2007.
Feyling-Hanssen, R. W. and Ulleberg, K.: A Tertiary-Quaternary section at Sarsbukta, Spitsbergen, Svalbard, and its foraminifera, Pol. Res., 2, 77–106, https://doi.org/10.1111/j.1751-8369.1984.tb00487.x, 1984.
Fischer, T., Hrubcová, P., Dahm, T., Woith, H., Vylita, T., Ohrnberger, M., Vlček, J., Horálek, J., Dědeček, P., Zimmer, M., Lipus, M. P., Pierdominici, S., Kallmeyer, J., Krüger, F., Hannemann, K., Korn, M., Kämpf, H., Reinsch, T., Klicpera, J., Vollmer, D., and Daskalopoulou, K.: ICDP drilling of the Eger Rift observatory: magmatic fluids driving the earthquake swarms and deep biosphere, Sci. Dril., 31, 31–49, https://doi.org/10.5194/sd-31-31-2022, 2022.
Foster, W. J., Danise, S., and Twitchett, R. J.: A silicified Early Triassic marine assemblage from Svalbard, J. Syst. Palaeontol., 15, 851–877, https://doi.org/10.1080/14772019.2016.1245680, 2017.
Foster, W. J., Asatryan, G., Botting, J., Rauzi, S., Lazarus, D. B., Buchwald, S. Z., Isson, T., Renaudie, J., and Kiessling, W.: Response of siliceous marine organisms to Permian-Triassic climate crisis based on new findings from central Spitsbergen, Svalbard, ESS Open Archive, https://doi.org/10.22541/essoar.169447472.26881234/v1, 2023.
Gastaldo, R. A., DiMichele, W. A., and Pfefferkorn, H. W.: Out of the icehouse into the greenhouse: a late Paleozoic analogue for modern global vegetational change, GSA today, https://rock.geosociety.org/net/gsatoday/archive/6/10/pdf/gt9610.pdf(last access: 4 October 2023), 1996.
Grundvåg, S. A., Helland-Hansen, W., Johannessen, E. P., Olsen, A. H., and Stene, S. A.: The depositional architecture and facies variability of shelf deltas in the Eocene Battfjellet Formation, Nathorst Land, Spitsbergen, Sedimentology, 61, 2172–2204, 2014.
Gutjahr, M., Ridgwell, A., Sexton, P. F., Anagnostou, E., Pearson, P. N., Pälike, H., Norris, R. D., Thomas, E., and Foster, G. L.: Very large release of mostly volcanic carbon during the Palaeocene–Eocene Thermal Maximum, Nature, 548, 573–577, https://doi.org/10.1038/nature23646, 2017.
Harding, I. C., Charles, A. J., Marshall, J. E. A., Pälike, H., Roberts, A. P., Wilson, P. A., Jarvis, E., Thorne, R., Morris, E., Moremon, R., Pearce, R. B., and Akbari, S.: Sea-level and salinity fluctuations during the Paleocene–Eocene thermal maximum in Arctic Spitsbergen, Earth Planet. Sc. Lett., 303, 97–107, https://doi.org/10.1016/j.epsl.2010.12.043, 2011.
Heimdal, T. H., Callegaro, S., Svensen, H. H., Jones, M. T., Pereira, E., and Planke, S.: Evidence for magma–evaporite interactions during the emplacement of the Central Atlantic Magmatic Province (CAMP) in Brazil, Earth Planet. Sc. Lett., 506, 476–492, https://doi.org/10.1016/j.epsl.2018.11.018, 2019.
Helland-Hansen, W. and Grundvåg, S. A.: The Svalbard Eocene-Oligocene (?) Central Basin succession: Sedimentation patterns and controls, Basin Res., 33, 729–753, 2021.
Herrle, J. O., Schröder-Adams, C. J., Davis, W., Pugh, A. T., Galloway, J. M., and Fath, J.: Mid-Cretaceous High Arctic stratigraphy, climate, and oceanic anoxic events, Geology, 43, 403–406, 2015.
Hesselbo, S. P., Gröcke, D. R., Jenkyns, H. C., Bjerrum, C. J., Farrimond, P., Morgans Bell, H. S., and Green, O. R.: Massive dissociation of gas hydrate during a Jurassic oceanic anoxic event, Nature, 406, 392–395, 2000.
Hoffman, P. F. and Schrag, D. P.: The snowball Earth hypothesis: testing the limits of global change, Terra Nova, 14, 129–155, https://doi.org/10.1046/j.1365-3121.2002.00408.x, 2002.
Holland, M. M. and Bitz, C. M.: Polar amplification of climate change in coupled models, Clim. Dynam., 21, 221–232, https://doi.org/10.1007/s00382-003-0332-6, 2003.
Hossain, A., Knorr, G., Jokat, W., and Lohmann, G.: Opening of the Fram Strait led to the establishment of a modern-like three-layer stratification in the Arctic Ocean during the Miocene, Arktos, 7, 1–12, https://doi.org/10.1007/s41063-020-00079-8, 2021.
Hutchinson, D. K., Coxall, H. K., O'Regan, M., Nilsson, J., Caballero, R., and de Boer, A. M.: Arctic closure as a trigger for Atlantic overturning at the Eocene-Oligocene Transition, Nat. Commun., 10, 3797, https://doi.org/10.1038/s41467-019-11828-z, 2019.
Hutchinson, D. K., Coxall, H. K., Lunt, D. J., Steinthorsdottir, M., de Boer, A. M., Baatsen, M., von der Heydt, A., Huber, M., Kennedy-Asser, A. T., Kunzmann, L., Ladant, J.-B., Lear, C. H., Moraweck, K., Pearson, P. N., Piga, E., Pound, M. J., Salzmann, U., Scher, H. D., Sijp, W. P., Śliwińska, K. K., Wilson, P. A., and Zhang, Z.: The Eocene–Oligocene transition: a review of marine and terrestrial proxy data, models and model–data comparisons, Clim. Past, 17, 269–315, https://doi.org/10.5194/cp-17-269-2021, 2021.
Inglis, G. N., Bragg, F., Burls, N. J., Cramwinckel, M. J., Evans, D., Foster, G. L., Huber, M., Lunt, D. J., Siler, N., Steinig, S., Tierney, J. E., Wilkinson, R., Anagnostou, E., de Boer, A. M., Dunkley Jones, T., Edgar, K. M., Hollis, C. J., Hutchinson, D. K., and Pancost, R. D.: Global mean surface temperature and climate sensitivity of the early Eocene Climatic Optimum (EECO), Paleocene–Eocene Thermal Maximum (PETM), and latest Paleocene, Clim. Past, 16, 1953–1968, https://doi.org/10.5194/cp-16-1953-2020, 2020.
Isbell, J. L., Fraiser, M. L., and Henry, L. C.: Examining the Complexity of Environmental Change during the Late Paleozoic and Early Mesozoic, PALAIOS, 23, 267–269, https://doi.org/10.2110/palo.2008.S03, 2008.
Jakobsson, M., Mayer, L., Coakley, B., Dowdeswell, J. A., Forbes, S., Fridman, B., Hodnesdal, H., Noormets, R., Pedersen, R., Rebesco, M., Schenke, H. W., Zarayskaya, Y., Accettella, D., Armstrong, A., Anderson, R. M., Bienhoff, P., Camerlenghi, A., Church, I., Edwards, M., Gardner, J. V., Hall, J. K., Hell, B., Hestvik, O., Kristoffersen, Y., Marcussen, C., Mohammad, R., Mosher, D., Nghiem, S. V., Pedrosa, M. T., Travaglini, P. G., and Weatherall, P.: The International Bathymetric Chart of the Arctic Ocean (IBCAO) Version 3.0, Geophys. Res. Lett., 39, L12609, https://doi.org/10.1029/2012GL052219, 2012.
Jelby, M. E., Śliwińska, K. K., Koevoets, M. J., Alsen, P., Vickers, M. L., Olaussen, S., and Stemmerik, L.: Arctic reappraisal of global carbon-cycle dynamics across the Jurassic–Cretaceous boundary and Valanginian Weissert Event, Palaeogeogr. Palaeocl., 555, 109847, https://doi.org/10.1016/j.palaeo.2020.109847, 2020.
Jin, Y., Wang, Y., Wang, W., Shang, Q., Cao, C., and Erwin, D. H.: Pattern of marine mass extinction near the Permian-Triassic boundary in South China, Science, 289, 432–436, 2000.
Johannessen, E. P., Henningsen, T., Bakke, N. E., Johansen, T. A., Ruud, B. O., Riste, P., Elvebakk, H., Jochmann, M., Elvebakk, G., and Woldengen, M. S.: Palaeogene clinoform succession on Svalbard expressed in outcrops, seismic data, logs and cores, First Break, 29, 35–44, 2011.
Jones, M. T., Jerram, D. A., Svensen, H. H., and Grove, C.: The effects of large igneous provinces on the global carbon and sulphur cycles, Palaeogeogr. Palaeocl., 441, 4–21, https://doi.org/10.1016/j.palaeo.2015.06.042, 2016.
Klausen, T. G., Nyberg, B., and Helland-Hansen, W.: The largest delta plain in Earth's history, Geology, 47, 470–474, https://doi.org/10.1130/G45507.1, 2019.
Koevoets, M. J., Abay, T. B., Hammer, Ø., and Olaussen, S.: High-resolution organic carbon–isotope stratigraphy of the Middle Jurassic–Lower Cretaceous Agardhfjellet Formation of central Spitsbergen, Svalbard, Palaeogeogr. Palaeocl., 449, 266–274, https://doi.org/10.1016/j.palaeo.2016.02.029, 2016.
Koppers, A., and Coggon, R.: Exploring earth by scientific ocean drilling: 2050 Science Framework, International Ocean Drilling Programme, https://www.iodp.org/2050-science-framework (last access: 4 October 2023), 2020.
Kristoffersen, Y., Ohta, Y., and Hall, J. K.: On the the origin of the Yermak Plateau north of Svalbard, Arctic Ocean, Norw. J. Geol./Norsk Geologisk Forening, 100, 202006, https://doi.org/10.17850/njg100-1-5, 2020.
Lee, S., Shi, G. R., Nakrem, H. A., Woo, J., and Tazawa, J.-I.: Mass extinction or extirpation: Permian biotic turnovers in the northwestern margin of Pangea, GSA Bulle., 134, 2399–2414, https://doi.org/10.1130/b36227.1, 2022.
Lorenz, H., Rosberg, J.-E., Juhlin, C., Klonowska, I., Lescoutre, R., Westmeijer, G., Almqvist, B. S. G., Anderson, M., Bertilsson, S., Dopson, M., Kallmeyer, J., Kück, J., Lehnert, O., Menegon, L., Pascal, C., Rejkjær, S., and Roberts, N. N. W.: COSC-2 – drilling the basal décollement and underlying margin of palaeocontinent Baltica in the Paleozoic Caledonide Orogen of Scandinavia, Sci. Dril., 30, 43–57, https://doi.org/10.5194/sd-30-43-2022, 2022.
Lourens, L. J., Sluijs, A., Kroon, D., Zachos, J. C., Thomas, E., Röhl, U., Bowles, J., and Raffi, I.: Astronomical pacing of late Palaeocene to early Eocene global warming events, Nature, 435, 1083–1087, https://doi.org/10.1038/nature03814, 2005.
Lucchi, R. G., St. John, K., and Ronge, T. A.: Expedition 403 Scientific Prospectus: Eastern Fram Strait Paleo-Archive (FRAME), International Ocean Discovery Program, https://doi.org/10.14379/iodp.sp.403.2023, 2023.
Lundschien, B. A., Mattingsdal, R., Johansen, S. K., and Knutsen, S.-M.: North Barents Composite Tectono-Sedimentary Element, Geological Society, London, Memoirs, 57, M57–2021-2039, https://doi.org/10.1144/M57-2021-39, 2024.
Midtkandal, I., Svensen, H. H., Planke, S., Corfu, F., Polteau, S., Torsvik, T. H., Faleide, J. I., Grundvåg, S.-A., Selnes, H., and Kürschner, W.: The Aptian (Early Cretaceous) oceanic anoxic event (OAE1a) in Svalbard, Barents Sea, and the absolute age of the Barremian-Aptian boundary, Palaeogeogr., Palaeocl., 463, 126–135, https://doi.org/10.1016/j.palaeo.2016.09.023, 2016.
Montañez, I. P. and Poulsen, C. J.: The Late Paleozoic ice age: an evolving paradigm, Annu. Rev. Earth Pl. Sc., 41, 629–656, 2013.
Montañez, I. P., Tabor, N. J., Niemeier, D., DiMichele, W. A., Frank, T. D., Fielding, C. R., Isbell, J. L., Birgenheier, L. P., and Rygel, M. C.: CO2-forced climate and vegetation instability during Late Paleozoic deglaciation, Science, 315, 87–91, 2007.
Mørk, A., Embry, A. F., and Weitschat, W.: Triassic transgressive-regressive cycles in the Sverdrup Basin, Svalbard and the Barents Shelf, in: Correlation in Hydrocarbon Exploration, edited by: Collinson, J. D., Springer, Dordrecht, https://doi.org/10.1007/978-94-009-1149-9_11, 1989.
Mørk, A., Dallmann, W., Dypvik, H., Johannessen, E., Larssen, G., Nagy, J., Nøttvedt, A., Olaussen, S., Pchelina, T., and Worsley, D.: Mesozoic lithostratigraphy, Lithostratigraphic lexicon of Svalbard. Upper Palaeozoic to Quaternary bedrock. Review and recommendations for nomenclature use, Norwegian Polar Institute, 127–214, ISBN 82-7666-166-1, 1999.
Nakrem, H. A. and Mørk, A.: New early Triassic Bryozoa (Trepostomata) from Spitsbergen, with some remarks on the stratigraphy of the investigated horizons, Geol. Mag., 128, 129–140, https://doi.org/10.1017/S001675680001832X, 1991.
Ogg, J. G., Ogg, G. M., and Gradstein, F. M.: A concise geologic time scale: 2016, Elsevier, ISBN 9780444637710, 2016.
Ohm, S. E., Larsen, L., Olaussen, S., Senger, K., Birchall, T., Demchuk, T., Hodson, A., Johansen, I., Titlestad, G. O., and Karlsen, D. A.: Discovery of shale gas in organic-rich Jurassic successions, Adventdalen, Central Spitsbergen, Norway, Norsk Geol. Tidsskr., 99, 349–376, https://doi.org/10.17850/njg007, 2019.
Olaussen, S., Senger, K., Braathen, A., Grundvåg, S. A., and Mørk, A.: You learn as long as you drill; research synthesis from the Longyearbyen CO2 Laboratory, Svalbard, Norway, Norw. J. Geol., 99, 157–187, https://doi.org/10.17850/njg008, 2019.
Olaussen, S., Grundvåg, S.-A., Senger, K., Anell, I., Betlem, P., Birchall, T., Braathen, A., Dallmann, W., M., J., Johannessen, E. P., Lord, G., Mørk, A., Osmundsen, P. T., Smyrak-Sikora, A., and Stemmerik, L.: Svalbard Composite Tectono-Stratigraphic Element, High Arctic Norway Geological Society of London Memoirs, https://doi.org/10.1144/M57-2021-36, 2023.
Penn, J. L., Deutsch, C., Payne, J. L., and Sperling, E. A.: Temperature-dependent hypoxia explains biogeography and severity of end-Permian marine mass extinction, Science, 362, eaat1327, https://doi.org/10.1126/science.aat1327, 2018.
Percival, L., Tedeschi, L. R., Creaser, R., Bottini, C., Erba, E., Giraud, F., Svensen, H., Savian, J., Trindade, R., and Coccioni, R.: Determining the style and provenance of magmatic activity during the Early Aptian Oceanic Anoxic Event (OAE 1a), Global Planet. Change, 200, 103461, https://doi.org/10.1016/j.gloplacha.2021.103461, 2021.
Pérez, L. F., Knutz, P. C., Hopper, J., Seidenkrantz, M.-S., and O'Regan, M.: Scientific drilling in Northeast Greenland: Greenland Ice Sheet sensitivity to polar amplification and long-term ice-ocean-tectonic interactions, EGU General Assembly 2023, Vienna, Austria, 24–28 April 2023, EGU23-11575, https://doi.org/10.5194/egusphere-egu23-11575, 2023.
Planke, S., Berndt, C., Alvarez Zarikian, C. A., and Expedition 396 Scientists: Proceedings of the International Ocean Discovery Program Volume 396 – Mid-Norwegian Margin Magmatism and Paleoclimate Implications, International Ocean Discovery Program, https://doi.org/10.14379/iodp.proc.396.2023, 2023.
Poirier, A. and Hillaire-Marcel, C.: Improved Os-isotope stratigraphy of the Arctic Ocean, Geophys. Res. Lett., 38, L14607, https://doi.org/10.1029/2011GL047953, 2011.
Price, G. D., Bajnai, D., and Fiebig, J.: Carbonate clumped isotope evidence for latitudinal seawater temperature gradients and the oxygen isotope composition of Early Cretaceous seas, Palaeogeogr. Palaeocl., 552, 109777, https://doi.org/10.1016/j.palaeo.2020.109777, 2020.
Rodríguez-Tovar, F., Dorador, J., Zuchuat, V., Planke, S., and Hammer, Ø.: Response of macrobenthic trace maker community to the end-Permian mass extinction in Central Spitsbergen, Svalbard, Palaeogeogr. Palaeocl., 581, 110637, https://doi.org/10.1016/j.palaeo.2021.110637, 2021.
Rooney, A. D., Strauss, J. V., Brandon, A. D., and Macdonald, F. A.: A Cryogenian chronology: Two long-lasting synchronous Neoproterozoic glaciations, Geology, 43, 459–462, 2015.
Ruhl, M. and Kürschner, W. M.: Multiple phases of carbon cycle disturbance from large igneous province formation at the Triassic-Jurassic transition, Geology, 39, 431–434, https://doi.org/10.1130/g31680.1, 2011.
Sangiorgi, F., Brumsack, H. J., Willard, D. A., Schouten, S., Stickley, C. E., O'Regan, M., Reichart, G. J., Sinninghe Damsté, J. S., and Brinkhuis, H.: A 26 million year gap in the central Arctic record at the greenhouse-icehouse transition: Looking for clues, Paleoceanography, 23, PA1S04, https://doi.org/10.1029/2007PA001477, 2008.
Schaaf, N. W., Osmundsen, P. T., Van der Lelij, R., Schönenberger, J., Lenz, O. K., Redfield, T. F., and Senger, K.: Tectono-sedimentary evolution of the eastern Forlandsundet Graben, Svalbard, Norw. J. Geol., 100, 202021, https://doi.org/10.17850/njg100-4-4, 2021.
Schlanger, S. O. and Jenkyns, H.: Cretaceous oceanic anoxic events: causes and consequences, Geol. Mijnbouw, 55, 179–184, 1976.
Schobben, M., Foster, W. J., Sleveland, A. R., Zuchuat, V., Svensen, H. H., Planke, S., Bond, D. P., Marcelis, F., Newton, R. J., and Wignall, P. B.: A nutrient control on marine anoxia during the end-Permian mass extinction, Nat. Geosci., 13, 640–646, https://doi.org/10.1038/s41561-020-0622-1, 2020.
Senger, K., Brugmans, P., Grundvåg, S.-A., Jochmann, M. M., Nøttvedt, A., Olaussen, S., Skotte, A., and Smyrak-Sikora, A.: Petroleum, coal and research drilling onshore Svalbard: a historical perspective, Norw. J. Geol., 99, 30 pp., https://doi.org/10.17850/njg99-3-1, 2019.
Senger, K., Betlem, P., Birchall, T., Gonzaga Jr, L., Grundvåg, S.-A., Horota, R. K., Laake, A., Kuckero, L., Mørk, A., and Planke, S.: Digitising Svalbard's Geology: the Festningen Digital Outcrop Model, First Break, 40, 47–55, 2022.
Sluijs, A., Frieling, J., Inglis, G. N., Nierop, K. G. J., Peterse, F., Sangiorgi, F., and Schouten, S.: Late Paleocene–early Eocene Arctic Ocean sea surface temperatures: reassessing biomarker paleothermometry at Lomonosov Ridge, Clim. Past, 16, 2381–2400, https://doi.org/10.5194/cp-16-2381-2020, 2020.
Smyrak-Sikora, A., Nicolaisen, J. B., Braathen, A., Johannessen, E. P., Olaussen, S., and Stemmerik, L.: Impact of growth faults on mixed siliciclastic-carbonate-evaporite deposits during rift climax and reorganisation – Billefjorden Trough, Svalbard, Norway, Basin Res., 33, 2643–2674, https://doi.org/10.1111/bre.12578, 2021.
Soreghan, G. S., Beccaletto, L., Benison, K. C., Bourquin, S., Feulner, G., Hamamura, N., Hamilton, M., Heavens, N. G., Hinnov, L., Huttenlocker, A., Looy, C., Pfeifer, L. S., Pochat, S., Sardar Abadi, M., Zambito, J., and the Deep Dust workshop participants: Report on ICDP Deep Dust workshops: probing continental climate of the late Paleozoic icehouse–greenhouse transition and beyond, Sci. Dril., 28, 93–112, https://doi.org/10.5194/sd-28-93-2020, 2020.
Sorento, T., Olaussen, S., and Stemmerik, L.: Controls on deposition of shallow marine carbonates and evaporites – lower Permian Gipshuken Formation, central Spitsbergen, Arctic Norway, Sedimentology, 67, 207–238, https://doi.org/10.1111/sed.12640, 2020.
Steel, R., Gjelberg, J., Helland-Hansen, W., Kleinspehn, K., Nøttvedt, A., and Rye-Larsen, M.: The Tertiary strike-slip basins and orogenic belt of Spitsbergen, in: Strike-Slip Deformation, Basin Formation, and Sedimentation, edited by: Biddle, K. T. and Christie-Blick, N., SEPM Society for Sedimentary Geology, https://doi.org/10.2110/pec.85.37.0319, 1985.
Steel, R. J. and Worsley, D.: Svalbard's post-Caledonian strata – an atlas of sedimentational patterns and palaeogeographic evolution, in: Petroleum Geology of the North European Margin, edited by: Spencer, A. M., Graham & Trotman, London, 109–135, https://doi.org/10.1007/978-94-009-5626-1_9, 1984.
Stickley, C. E., Brinkhuis, H., Schellenberg, S. A., Sluijs, A., Röhl, U., Fuller, M., Grauert, M., Huber, M., Warnaar, J., and Williams, G. L.: Timing and nature of the deepening of the Tasmanian Gateway, Paleoceanography, 19, PA4027, https://doi.org/10.1029/2004PA001022, 2004.
Stickley, C. E., St John, K., Koç, N., Jordan, R. W., Passchier, S., Pearce, R. B., and Kearns, L. E.: Evidence for middle Eocene Arctic sea ice from diatoms and ice-rafted debris, Nature, 460, 376–379, https://doi.org/10.1038/nature08163, 2009.
Straume, E. O., Nummelin, A., Gaina, C., and Nisancioglu, K. H.: Climate transition at the Eocene–Oligocene influenced by bathymetric changes to the Atlantic–Arctic oceanic gateways, P. Natl. Acad. Sci. USA, 119, e2115346119, https://doi.org/10.1073/pnas.2115346119, 2022.
Suan, G., Popescu, S.-M., Suc, J.-P., Schnyder, J., Fauquette, S., Baudin, F., Yoon, D., Piepjohn, K., Sobolev, N. N., and Labrousse, L.: Subtropical climate conditions and mangrove growth in Arctic Siberia during the early Eocene, Geology, 45, 539–542, https://doi.org/10.1130/G38547.1, 2017.
Svensen, H., Planke, S., Malthe-Sørenssen, A., Jamtveit, B., Myklebust, R., Rasmussen Eidem, T., and Rey, S. S.: Release of methane from a volcanic basin as a mechanism for initial Eocene global warming, Nature, 429, 542–545, 2004.
Svensen, H., Planke, S., Polozov, A. G., Schmidbauer, N., Corfu, F., Podladchikov, Y. Y., and Jamtveit, B.: Siberian gas venting and the end-Permian environmental crisis, Earth Planet. Sc. Lett., 277, 490–500, https://doi.org/10.1016/j.epsl.2008.11.015, 2009.
Toggweiler, J. and Bjornsson, H.: Drake Passage and palaeoclimate, J. Quaternary Sci., 15, 319–328, 2000.
Torsvik, T. H. and Cocks, L. R. M.: The integration of palaeomagnetism, the geological record and mantle tomography in the location of ancient continents, Geol. Mag., 156, 242–260, 2019.
Tripati, A. and Darby, D.: Evidence for ephemeral middle Eocene to early Oligocene Greenland glacial ice and pan-Arctic sea ice, Nat. Commun., 9, 1038, https://doi.org/10.1038/s41467-018-03180-5, 2018.
Vickers, M. L., Jelby, M. E., Śliwińska, K. K., Percival, L. M., Wang, F., Sanei, H., Price, G. D., Ullmann, C. V., Grasby, S. E., and Reinhardt, L.: Volcanism and carbon cycle perturbations in the High Arctic during the Late Jurassic–Early Cretaceous, Palaeogeogr. Palaeocl., 613, 111412, https://doi.org/10.1016/j.palaeo.2023.111412, 2023.
Vigran, J. O., Mangerud, G., Mørk, A., Worsley, D., and Hochuli, P. A.: Palynology and geology of the Triassic succession of Svalbard and the Barents Sea, Norges geologiske undersokelse, Geological Survey of Norway Special Publication, 14, ISBN 978-82-7385-156-7, 2014.
von Appen, W.-J., Schauer, U., Somavilla, R., Bauerfeind, E., and Beszczynska-Möller, A.: Exchange of warming deep waters across Fram Strait, Deep-Sea Res. Pt. I, 103, 86–100, https://doi.org/10.1016/j.dsr.2015.06.003, 2015.
Weger, R. J., Eberli, G. P., Rodriguez Blanco, L., Tenaglia, M., and Swart, P. K.: Finding a VOICE in the Southern Hemisphere: A new record of global organic carbon?, Geol. Soc. Am. Bull., 135, 2107–2120, https://doi.org/10.1130/B36405.1, 2022.
Weijers, J. W., Schouten, S., Sluijs, A., Brinkhuis, H., and Damsté, J. S. S.: Warm arctic continents during the Palaeocene–Eocene thermal maximum, Earth Planet. Sc. Lett., 261, 230–238, https://doi.org/10.1016/j.epsl.2007.06.033, 2007.
West, C. K., Greenwood, D. R., and Basinger, J. F.: Was the Arctic Eocene “rainforest” monsoonal? Estimates of seasonal precipitation from early Eocene megafloras from Ellesmere Island, Nunavut, Earth Planet. Sc. Lett., 427, 18–30, https://doi.org/10.1016/j.epsl.2015.06.036, 2015.
Westerhold, T., Marwan, N., Drury, A. J., Liebrand, D., Agnini, C., Anagnostou, E., Barnet, J. S., Bohaty, S. M., De Vleeschouwer, D., and Florindo, F.: An astronomically dated record of Earth's climate and its predictability over the last 66 million years, Science, 369, 1383–1387, https://doi.org/10.1126/science.aba6853, 2020.
Wignall, P., Morante, R., and Newton, R.: The Permo-Triassic transition in Spitsbergen: δ13Corg chemostratigraphy, Fe and S geochemistry, facies, fauna and trace fossils, Geol. Mag., 135, 47–62, https://doi.org/10.1017/S0016756897008121, 1998.
Willard, D. A., Donders, T. H., Reichgelt, T., Greenwood, D. R., Sangiorgi, F., Peterse, F., Nierop, K. G., Frieling, J., Schouten, S., and Sluijs, A.: Arctic vegetation, temperature, and hydrology during Early Eocene transient global warming events, Global Planet. Change, 178, 139–152, https://doi.org/10.1016/j.gloplacha.2019.04.012, 2019.
Zachos, J. C., Dickens, G. R., and Zeebe, R. E.: An early Cenozoic perspective on greenhouse warming and carbon-cycle dynamics, Nature, 451, 279–283, https://doi.org/10.1038/nature06588, 2008.
Zuchuat, V., Sleveland, A. R. N., Twitchett, R. J., Svensen, H. H., Turner, H., Augland, L. E., Jones, M. T., Hammer, Ø., Hauksson, B. T., Haflidason, H., Midtkandal, I., and Planke, S.: A new high-resolution stratigraphic and palaeoenvironmental record spanning the End-Permian Mass Extinction and its aftermath in central Spitsbergen, Svalbard, Palaeogeogr. Palaeocl., 554, 109732, https://doi.org/10.1016/j.palaeo.2020.109732, 2020.
- Abstract
- Introduction
- Previous onshore drilling on Svalbard: a brief synthesis
- Identified scientific drilling targets
- From vision to drilling
- Synergies to ongoing ICDP and IODP projects
- Outcomes and future plans
- Appendix A
- Appendix B: Abbreviations
- Data availability
- Author contributions
- Competing interests
- Disclaimer
- Acknowledgements
- Financial support
- Review statement
- References
- Abstract
- Introduction
- Previous onshore drilling on Svalbard: a brief synthesis
- Identified scientific drilling targets
- From vision to drilling
- Synergies to ongoing ICDP and IODP projects
- Outcomes and future plans
- Appendix A
- Appendix B: Abbreviations
- Data availability
- Author contributions
- Competing interests
- Disclaimer
- Acknowledgements
- Financial support
- Review statement
- References