the Creative Commons Attribution 4.0 License.
the Creative Commons Attribution 4.0 License.
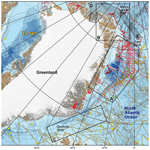
NorthGreen: unlocking records from sea to land in Northeast Greenland
Paul C. Knutz
John R. Hopper
Marit-Solveig Seidenkrantz
Matt O'Regan
Stephen Jones
The increasing anthropogenic CO2 forcing of the climate system calls for a better understanding of how polar ice sheets may respond to accelerating global warming. The sensitivity of the Greenland ice sheet to polar amplification, changes in ocean heat transport, and deteriorating perennial sea ice conditions makes the Northeast Greenland margin a pertinent location with respect to understanding the impact of climate change on ice sheet instability and associated sea level rise. Throughout the Cenozoic, ocean heat fluxes toward and along Northeast Greenland have been controlled by water mass exchanges between the Arctic and Atlantic oceans. A key element here is the current flow through oceanic gateways, notably the Fram Strait and the Greenland–Scotland Ridge. To gain a long-term (million-year) perspective of ice sheet variability in this region, it is essential to understand the broader context of ice–ocean–tectonic interactions. Coupling between the ice sheet, the subsurface, the ocean, and sea ice are readily observable today in Northeast Greenland, but geological records to illuminate long-term trends and their interplay with other parts of the global climate system are lacking. Consequently, the NorthGreen workshop was organized by the Geological Survey of Denmark and Greenland in collaboration with Aarhus (Denmark) and Stockholm (Sweden) universities in November 2022 to develop mission-specific platform (MSP) proposals for drilling the Northeast Greenland margin under the umbrella of the MagellanPlus Workshop Series Programme of the European Consortium for Ocean Research Drilling (ECORD). Seventy-one participants representing a broad scientific community discussed key scientific questions and primary targets that could be addressed through scientific drilling in Northeast Greenland. Three pre-proposals were initiated during the workshop targeting Morris Jesup Rise, the Northeast Greenland continental shelf, and Denmark Strait.
- Article
(7393 KB) - Full-text XML
- BibTeX
- EndNote
The Greenland ice sheet is km2 with a maximum thickness of 3 km. During the past few decades, it has been a major contributor to global sea level rise (van den Broeke et al., 2016; Shepherd et al., 2020; Christ et al., 2023), and if melted completely, the Greenland ice sheet would represent a sea level rise equivalent to ∼ 7.5 m (e.g., Dutton et al., 2015; Morlighem et al., 2017). Numerical models suggest a present negative ice mass balance and a marked increase in ice sheet runoff as a consequence of polar amplification in the Arctic (Rignot et al., 2011; Golledge et al., 2019; Hofer et al., 2020). In addition, sea ice cover in the Arctic Ocean has substantially shrunk over the last few years, with ice models suggesting a high probability of an ice-free Arctic Ocean in late summer by 2050 (Notz and SIMIP Community, 2020). However, predictive models on extended time frames are often working outside of the limits in which they have demonstrable skill. The projections of future scenarios under the current trend of global climate change demand a better understanding of long-term ice–ocean–tectonic interactions (IPCC, 2019). This broad achievement requires access to paleodata that are hidden in the sedimentary successions of polar margins.
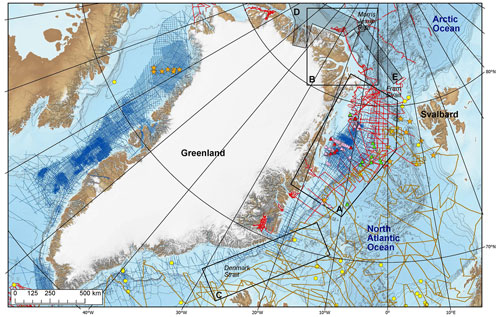
Figure 1Greenland margin's bathymetry map from the GEBCO_2019 grid (http://www.gebco.net, last access: 24 July 2023) showing the available data as follows: blue lines – commercial seismic reflection data available at the Geological Survey of Denmark and Greenland (GEUS); red lines – other seismic reflection data available from academic data owners; brown lines – single-channel seismic data; yellow dots – existing Deep Sea Drilling Project (DSDP) and ODP drill sites; orange stars – proposed IODP drill sites with JOIDES Resolution Facility Board (JRFB); red triangles – Kanumas Consortium 2008 cores; pink triangles – other industry gravity cores; and green triangles – industry dredges. Cores only reaching through the Holocene are not shown. A, B, and C denote areas of interest for the NorthGreen MagellanPlus workshop; D and E denote GEOEO and GoNorth planned expeditions in 2024.
Antarctic and Southern Ocean marine sediments have been recovered on seven scientific drilling expeditions under the framework of the International Ocean Discovery Program (IODP) and its predecessors. These Cenozoic marine sediment archives have significantly contributed to the understanding of the sensitivity of the Antarctic ice sheet to elevated global temperatures and greenhouse gas concentrations (Escutia et al., 2019). However, scientific drilling expeditions in the Arctic and northern North Atlantic oceans have, until now, focused on sites far from the Greenland margins (Fig. 1), e.g., Ocean Drilling Program (ODP) 151, IODP 395, and IODP 302 (Arctic Coring Expedition – ACEX) as well as the scheduled IODP Expedition 403 to the eastern Fram Strait (Lucchi et al., 2023). The hitherto only drilling campaign directly targeting Greenland margin sedimentary successions has been IODP Expedition 400, implemented in 2023, which has investigated the glacial–interglacial variability and late-Cenozoic paleoceanography of the Northwest Greenland margin (Knutz et al., 2024). Thus, the Northeast Greenland glaciation history remains a major knowledge gap, hampering understanding of the influence of the Greenland ice sheet on future climate projections and of its sensitivity to a warmer-than-present climate. The aim of this work is to provide a general overview of the recent activities to foster scientific drilling in the Northeast Greenland Margin, based on the long-term research strategy discussed during the NorthGreen MagellanPlus workshop.
The Northeast Greenland margin experienced a long history of rifting prior to the onset of the continental breakup and oceanic spreading during the Paleocene–Eocene transition (e.g., Talwani and Eldholm, 1977; Hamann et al., 2005; Gaina et al., 2017). The post-breakup sedimentary record varies in thickness along the margin and comprises thick sedimentary wedges deposited during distinct phases of Neogene uplift (e.g., Hamann et al., 2005; Berger and Jokat, 2008, 2009; Tsikalas et al., 2012). However, glaciation-related processes have also left imprints on the margin's sedimentary sequences, recording the variability in sea ice, ice sheets, ice shelves, and glaciers on seasonal, interannual, and glacial–interglacial timescales. Evidence of a glaciated hinterland and the presence of tidewater glaciers in Greenland extends back to Eocene–Miocene times (e.g., Solheim et al., 1998; Tripati et al., 2008; Thiede et al., 2010). A permanent Greenland ice sheet was formed during the middle Miocene (Larsen et al., 1994; St. John and Krissek, 2002; Berger and Jokat, 2008, 2009; Thiede et al., 2010), eventually prograding asynchronously over the continental shelves (Døssing et al., 2016; Pérez et al., 2018; Heirman et al., 2019). The subsequent glacial history of Greenland has been marked by the dynamic behavior of the ice sheet, with several pulses of glacial intensification at 7, 2.8, 1.9, and 0.8 Ma (Larsen et al., 1994; Solheim et al., 1998; St. John and Krissek, 2002; Bierman et al., 2016). During periods of maximum ice sheet advance, trough-mouth fans formed at the paleo continental shelf edge as thick, prograding wedges of sediments delivered by the cross-shelf flow of ice streams (e.g., Pérez et al., 2018). The ice streams in Northeast Greenland are highly sensitive to warm climate periods. For example, the Northeast Greenland ice stream retreated up to 70 km behind its present-day extent during Quaternary periods of high orbital precession index and summer temperatures (Larsen et al., 2018).
Ice sheet dynamics in Greenland have been influenced by the variability in oceanographic patterns and, therefore, the opening and deepening of oceanic gateways (Smith and Pickering, 2003). The opening of the Fram Strait resulted in the onset of the modern, ventilated Arctic Ocean with the exchange of water masses with the northern North Atlantic Ocean (Jakobsson et al., 2007; Rudels, 2009). The timing of gateway formation is still debated (e.g., Engen et al., 2008; Ehlers and Jokat, 2013; Jokat et al., 2015), but outflow of cold water from the Arctic Ocean to the northern North Atlantic Ocean through the Fram Strait is generally thought to coincide with the onset of the East Greenland Current (EGC) during the late Miocene (Wolf and Thiede, 1991; Våge et al., 2013) or early Pliocene (De Schepper et al., 2015; Clotten et al., 2019). The southern flow of the EGC was also controlled by the opening of the Denmark Strait during the Miocene, although the more precise timing is debated, and subsequently influenced by the dynamic topography along the Greenland–Scotland Ridge in response to pulsing of the Iceland plume (White et al., 1995; Wright and Miller, 1996; Poore et al., 2006; Engen et al., 2008; Parnell-Turner et al., 2015). Major pre-Quaternary changes in the EGC are recorded by contourite deposits on the slopes of East Greenland (e.g., Davies et al., 2021). On the continental shelf, warm and saline water of Atlantic origin, the Atlantic Intermediate Water (AIW), enters major troughs (Budéus and Schneider, 1995) while Submarine Melt Water (SMW) flows eastwards to the deep basins, transforming the Atlantic Meridional Overturning Circulation (AMOC) (Böning et al., 2016).
A large portion of the aforementioned paleoclimate history is supported by data and samples from the Ocean Drilling Program (ODP) Expedition 151 and Expedition 162 in the central North Atlantic Ocean (Jansen et al., 1996; Thiede et al., 1996). However, the Northeast Greenland margin represents a major knowledge gap in the Cenozoic climatic–oceanographic–tectonic evolution of Earth, and key scientific uncertainties remain with respect to three major topics: sea ice history, Greenland ice sheet evolution, and oceanic gateways. With respect to sea ice history, the following question remains: “When did a perennial sea ice cover develop in the Arctic Ocean, and how often did sea-ice-free conditions occur?”. Regarding Greenland ice sheet evolution, the following questions are put forward: “When did glaciation in Greenland begin, and how did the ice sheet evolve over the Cenozoic?”, “Did past warm climates (e.g., super-interglacials, Eemian) cause whole or partial deglaciation in Northern Greenland (Schaefer et al., 2016; Christ et al., 2023)?”, and “Alternatively, is glaciation of Northeast Greenland a relatively pervasive feature in the climate system, with the ice sheet being preserved during peak warmings (Bierman et al., 2016)?”. Finally, with respect to oceanic gateways, the following questions remain: “What is the timing and progression of the opening of Fram Strait?”, “What is the subsidence history of the conjugate Morris Jesup Rise and Yermak Plateau?”, “How did their formation and subsidence influence the oceanographic development of the Arctic Ocean from isolated to fully ventilated (Jakobsson et al., 2007; Poirier and Hillaire-Marcel, 2011)?”, and “When did the Denmark Strait open, how was its cross-sectional area subsequently influenced by Icelandic mantle plume activity, and what were the relative roles of gateway tectonics and ice sheet/sea ice evolution in controlling the AMOC?”.
Paleorecords proximal to Greenland and across the land–ocean boundary can address these fundamental questions that have far-reaching implications for our understanding of how the climate system operates now as well as how it operated in the past. Data required to illuminate these questions are potentially contained in the sedimentary successions offshore and onshore in Northeast Greenland (Bennike et al., 2002, 2010). While harsh environmental conditions have limited the geophysical data acquisition needed to define drilling targets, data collection in these remote and ice-filled waters has increased substantially over the last 2 decades. The Atlantic margin of Northeast Greenland (Area A in Fig. 1) is now well covered by high-quality industry seismic data (Christiansen, 2021). In addition, the collection of shallow seismic data, gravity cores, and shallow industry boreholes demonstrates the possibility of mission-specific platform (MSP) operations. Hence, sufficient data are available to start developing a MSP proposal on the northeastern continental shelf (Area A). Although far less explored, new seismic data from icebreaker-led expeditions to the Arctic margin of North and Northeast Greenland (Area B in Fig. 1) as well as novel approaches to data collection from drifting ice stations (Kristoffersen et al., 2021) have revealed exciting new scientific questions and potential drilling targets that can be exploited using MSP drilling capabilities. Along the remote northern coast of Greenland, ice conditions have rapidly become more amenable in recent years, facilitating logistically feasible work, as proven by the 2018 I/B Polarstern expedition that collected deep seismic data north of Peary Land (Damm, 2019). Based on the data availability, the key objectives of the NorthGreen MagellanPlus workshop were as follows:
-
to discuss the potential development of coordinated IODP MSP proposals in Northeast Greenland including both onshore and offshore operations, within short and long time frames;
-
to define specific Cenozoic drilling targets based on existing data;
-
to identify the data gaps in key research areas, e.g., along shelf to fjord transects;
-
to establish collaborations among the wide scientific community by integrating the results of past and forthcoming IODP expeditions around the Greenland margins and expanding this scientific network.
4.1 Workshop attendance
The NorthGreen MagellanPlus workshop was held at GEUS in Copenhagen, Denmark, on 21–23 November 2022. The program included scientific and technical talks delivered by international keynote speakers, breakout sessions, and posters. Seventy-one participants represented a wide scientific community of marine scientists, paleoclimatologists, and terrestrial geologists and glaciologists (Fig. 2). The workshop included participants from 13 countries with 56 in-person and 15 online attendants (Table 1). Fifteen of the participants were early-career researchers. Thus, NorthGreen was a successful workshop that gathered an international, multidisciplinary group of scientists to discuss scientific objectives and hypotheses relevant for the Northeast Greenland margins and the adjoining ocean regions.
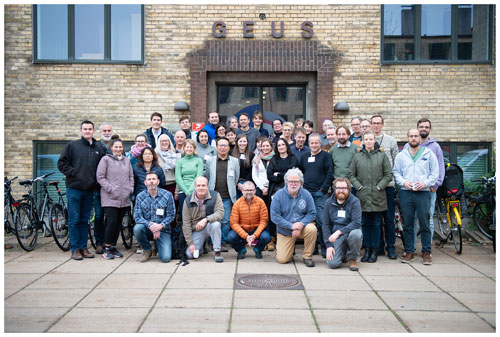
Figure 2In-person participants of the NorthGreen MagellanPlus workshop at GEUS in November 2022 in Copenhagen, Denmark. Photo by GEUS.
4.2 MSP proposals in Northeast Greenland
Scientific drilling of the Northeast Greenland margins allows research within all seven of the strategic objectives of the IODP 2050 Science Framework (Koppers and Coggon, 2020). In particular, the proposed objectives crucial for the Ground-Truthing Future Climate Change flagship initiative. Thus, the NorthGreen MagellanPlus workshop constituted the kickoff of a long-term (∼ 10 years) research strategy on the Northeast Greenland margins. During the workshop, key drilling areas were defined (Fig. 1). Highlighted topics were (i) the Greenland ice sheet extension during past interglacial periods; (ii) key Cenozoic intervals to provide paleo-constraints for future climate projections; (iii) climate and biosphere interactions; and (iv) oceanic gateways, specifically the Arctic–North Atlantic tectonic connections and their impact on global thermohaline circulation. The working teams and scientific objectives of three MSP proposals were outlined during the workshop.
4.2.1 Morris Jesup Rise: resurrecting an old pre-proposal
The Morris Jesup Rise (Area B in Fig. 1) extends ∼ 250 km into the central Arctic Ocean from the coast of northern Greenland. It is a conjugate feature to the Yermak Plateau with respect to the Gakkel Ridge (Fig. 3). These two physiographic features are believed to be of volcanic origin, formed at the triple junction between the Greenland, North American, and Eurasian plates. Estimates for the timing of volcanic emplacement vary widely, from the Late Cretaceous to Eocene–Oligocene times (Kristoffersen et al., 2021). The Morris Jesup Rise and Yermak Plateau were ultimately rifted apart by the propagation of seafloor spreading along the Gakkel Ridge between ∼ 33 and 25 Ma (Brozena et al., 2003; Gion et al., 2017), over 20 Myr after seafloor spreading began in the Eurasian Basin. The eventual opening of the Fram Strait had a profound impact on the oceanography of the Arctic and is marked in the ACEX record by a transition from poorly oxygenated to fully ventilated conditions (Jakobsson et al., 2007). New seismic data acquired from a floating ice station that crossed the Morris Jesup Rise in 2015 (Kristoffersen et al., 2016) have shown that a relatively thin drape (100 m) of Miocene/Pliocene to Present sediments overlay a peneplained unconformity that formed prior to the post-rifting subsidence of the Morris Jesup Rise (Kristoffersen et al., 2021). Constraining the timing and type of volcanic activity that formed the Morris Jesup Rise as well as its subsequent subsidence history would provide important new data for testing the current interpretation based on seafloor magnetic anomalies and for improving plate tectonic models on the development of the oceanic–continental region around northernmost Greenland.
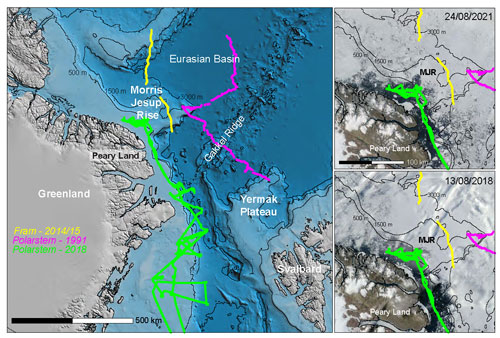
Figure 3Geographic setting of Morris Jesup Rise (MJR) and Yermak Plateau flanking the Fram Strait. Seismic data crossing the MJR exist from three previous cruises (shown by the colored lines). Bathymetry is from the International Bathymetric Chart of the Arctic Ocean v.3.0 (Jakobsson et al., 2012). The right-hand panels show satellite images that illustrate examples of how the sea ice cover over MJR and the northern Greenland margin broke up in August 2018 (bottom) and August 2021 (top). Georeferenced satellite images were downloaded from https://worldview.earthdata.nasa.gov/ (last access: 24 July 2023).
The Atlantic water that enters the Arctic Ocean through the eastern Fram Strait and across the Barents Sea continues a cyclonic circulation along the continental slopes of the inner Arctic Ocean and reenters the Norwegian–Greenland Sea after passing the Morris Jesup Rise. Therefore, sedimentary archives from the Morris Jesup Rise would provide an integrated picture of water masses as they exit the Arctic Ocean and complement past (ODP Leg 151) and planned (IODP Expedition 403) expeditions to the eastern Fram Strait. Furthermore, the Morris Jesup Rise lies at the eastern edge of what has recently been termed the “Last Ice Area” (Newton et al., 2021), a region that hosts the oldest, thickest, and most persistent sea ice cover in the Arctic, making it an ideal target to recover long-term proxy-based insights into the persistence of sea ice in the central Arctic Ocean as well as the provenance of ice-rafted material that is exiting the Arctic.
A previous pre-proposal for drilling on the Morris Jesup Rise was submitted in 2009 (756-Pre) and received favorable reviews by the Science Evaluation Panel of IODP. However, the lack of site survey data and concerns about proposing deep drilling sites in a region prone to harsh sea ice conditions stalled the development of a full proposal. The NorthGreen workshop served as a catalyst for the resurrection of the drilling initiative, largely in response to the increased feasibility of drilling due to the breakup of sea ice in the region, newly acquired seismic data illuminating exciting new scientific questions and drilling targets, and three upcoming expeditions to the area in 2024 and 2025 (by Sweden, Norway, and Germany) that can collect additional geophysical and coring data in support of a full drilling proposal (Areas D and E in Fig. 1). Thus, the two main scientific goals of drilling Morris Jesup Rise will be as follows:
-
refine the plate tectonic development of the oceanic–continental region around northernmost Greenland with a focus on the formation, rifting, and subsidence of the Morris Jesup Rise as well as the breakup between the Barents Sea and Northeast Greenland margin;
-
reconstruct the paleoceanographic evolution of the central Arctic Ocean, focusing on the inception and persistence of sea ice in the Last Ice Area, the fluxes and sources of water masses exiting the inner Arctic, and the Cenozoic dynamics of the northern Greenland and other circum-Arctic ice sheets.
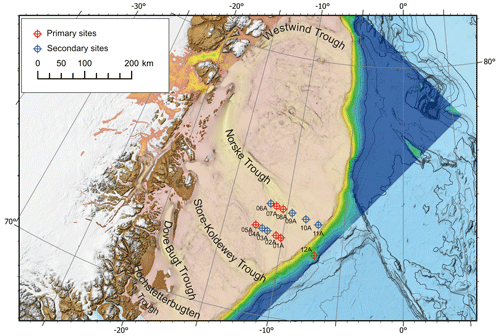
Figure 4Proposed drilling sites in IODP proposal 1011-Pre “Northeast Greenland Glaciated Margin” on the continental shelf and slope of Northeast Greenland. The high-resolution bathymetric map of Arndt et al. (2015) is overlaid on the International Bathymetric Chart of the Arctic Ocean v.3.0 (Jakobsson et al., 2012).
4.2.2 Northeast Greenland continental shelf: IODP 1011-Pre
The Greenland margin has the widest continental shelf along Northeast Greenland (Fig. 4), where the shelf edge is located up to ∼ 300 km from the coastline and gives way to the abyssal plain of the northern North Atlantic Ocean through a steep slope characterized by lobed morphology (Arndt et al., 2015). Internally, the Northeast Greenland continental shelf consists of major Paleozoic–Mesozoic basins formed during multiple phases of rifting that preceded the North Atlantic seafloor spreading around 56–54 Ma (Hamann et al., 2005; Stoker et al., 2016; Abdelmalak et al., 2023). Early volcanic activity and the occurrence of salt diapirs and hydrocarbons within the shelf basins have completed the margin structure (Hamann et al., 2005; Fyhn and Hopper, 2024). However, the Cenozoic history of Northeast Greenland has been marked by repeated erosion by ice streams emanating from the major fjords which subsequently coalesced offshore to form overdeepened cross-shelf troughs (Fig. 4).
The first emergence of a stable Greenland-wide ice sheet is usually related to the initial cooling phase of the Northern Hemisphere glaciation during the Quaternary (e.g., Bailey et al., 2013). Nevertheless, ephemeral ice sheets have been tracked back to the late Eocene (e.g., Maslin et al., 1998; Darby, 2014), and identified ice-rafted debris from ODP Sites 913 and 918 has been interpreted as originating from a permanent ice sheet covering east Greenland during the late Eocene and middle Miocene (e.g., Tripati et al., 2008; Thiede et al., 2010). However, both ODP sites are located on the abyssal plain away from the present-day Greenland coastline and, thus, do not record initial ice sheet inception. Determining the timing of the initial cross-shelf glaciation on Northeast Greenland would constrain the environmental conditions of the transition from land-based to marine-based ice sheets in the Northern Hemisphere.
The Northeast Greenland ice stream drains about 20 % of the Greenland ice sheet (Zwally et al., 2012). During previous glacial periods, carving offshore troughs, such as the Norske Trough (Fig. 4), led to the deposition of trough-mouth fans at the shelf edge (Fahnestock et al., 2001; Arndt et al., 2015). However, during warm interglacials, the warm water from the Return Atlantic Water reaches the inner continental shelf in Northeast Greenland, flowing through the shelf troughs (Straneo et al., 2017; Schaffer et al., 2020). Distinct climatic forcings have influenced the dynamics of the Greenland ice sheet, changing the role of the continental shelf troughs from ice-flow channels to warm-water conducts. Knowledge of the dynamics of the Greenland ice sheet during consecutive glacial–interglacial cycles is essential to understand its response to warm climates.
Following the NorthGreen workshop, IODP proposal 1011-Pre was submitted in April 2023 under the title “Northeast Greenland Glaciated Margin”. Due to positive feedback from the IODP Science Evaluation Panel, a full proposal is currently under development. The proposal focuses on the Greenland ice sheet as a tipping point within the Earth's climate system. Understanding the long-term stability of the Greenland ice sheet is critical for anticipating future climate and sea level scenarios, as the continental margin of Northeast Greenland constitutes a missing piece in this context (Area A in Fig. 1). The proposed drilling on the prograded shelf margin targets trough-mouth fans and buried contourite deposits as archives of the past evolution of the Greenland ice sheet. The highlighted objectives of the proposal are as follows: (1) to illuminate the timing and environmental conditions at the onset of glacial expansion, (2) to understand the dynamics of the Greenland ice sheet during abrupt changes in atmospheric and oceanographic conditions, and (3) to investigate the effects of regional tectonic and oceanographic changes on the polar cryosphere evolution. The drilling strategy consists of six primary sites complemented by six alternate sites along transects crossing the continental shelf and slope on the Norske Trough (Fig. 4). Discussions on further possibilities for onshore scientific drilling have been initiated. Several onshore projects lead by workshop participants have been highlighted as an initial step towards land-to-sea connections in Northeast Greenland (e.g., GreenDrill, 2023), with both a short-term and long-term perspective.
4.2.3 Denmark Strait gateway: DenGate proposal outline
DenGate is a recently formed international collaborative venture that focuses on paleoceanography and tectonic evolution of the Denmark Strait oceanic gateway (Fig. 5). The Denmark Strait, between Greenland and Iceland, and the Faroe–Iceland Ridge/Faroe–Shetland Basin, between Iceland and Scotland, are gateways within the Greenland–Scotland Ridge. The Greenland–Scotland Ridge is a shallow sill of thick oceanic crust that formed above the Icelandic mantle plume and constitutes an important barrier within the AMOC system, as it separates the main Atlantic Ocean from the Nordic Seas (and hence the Arctic Ocean). The Nordic Seas (or northern North Atlantic Ocean) are one of the two main global production sites of cold, deep ocean water. At present, the combined southward deepwater flow through the Denmark Strait and Faroe–Iceland Ridge gateways directly contributes about one-third of AMOC, and it indirectly influences the remainder (Hansen et al., 2004). The AMOC has fluctuated in strength considerably over timescales from decades to millions of years, and these changes correlate with changes in Northern Hemisphere climate (Rahmstorf, 2002). Community effort has been requested to resolve which components and pathways of the AMOC have altered, how, and why (Ceasar et al., 2021).
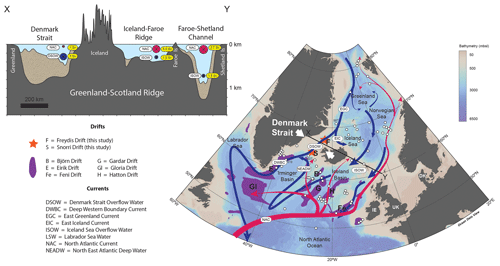
Figure 5Oceanographic context for the DenGate drilling proposal. Red and blue arrows indicate respective warm surface and cold deep ocean currents that comprise the northern hub of the Atlantic Meridional Overturning Circulation. Note that much of the sediment illustrated beneath the Denmark Strait section is derived from the continental shelf; the areal extents and thicknesses of the Snorri and Freydis drifts (orange stars) are unclear and require further seismic site survey. White circles show IODP, ODP, and DSDP sites. The data used to create the figure were sourced from the Ocean Data View software (Schlitzer, Reiner, Ocean Data View, http://odv.awi.de, 2023, last access: December 2023).
The age of the first opening of Denmark Strait is unclear. The Greenland–Scotland Ridge has been affected by a complicated series of oceanic spreading axis relocations ever since the breakup of Greenland and northwestern Europe in the earliest Eocene (Hardarson et al., 1997). It is not known whether the oceanic crust beneath the Denmark Strait was formed by a northward projection of the Reykjanes Ridge (the current plate boundary to the south of Iceland) or a southward projection of the Kolbeinsey Ridge (the current plate boundary to the north of Iceland). Thus, the age of the oceanic basement beneath the Denmark Strait is uncertain; consequently, there are large uncertainties in oceanic plate subsidence models with respect to its opening. Notwithstanding these uncertainties, some models predict that the Denmark Strait first opened during the Miocene, ∼ 20 to 10 Ma (Poore et al., 2006; Straume et al., 2020). Thus, there is considerable uncertainty regarding the role of the Denmark Strait in the EGC initiation. After its initial opening, subsidence of the Denmark Strait gateway was probably influenced by fluctuations in activity of the Icelandic mantle plume. Fluctuations in the accumulation of sediment drifts to the south of Iceland have been interpreted to mean that plume-driven vertical motions of the Denmark Strait and Faroe–Iceland Ridge gateways have influenced the AMOC (Parnell-Turner et al., 2015). This hypothesis was a main basis for the recent IODP Expedition 395. However, higher-frequency fluctuations in AMOC, including a dramatic weakening over the past century (Ceasar et al., 2021), cannot have been driven by tectonics and rather point to a complex relationship between AMOC, climate, and tectonics.
Direct measurements of deepwater flow through the Denmark Strait, termed Denmark Strait Overflow Water (DSOW), at high temporal resolution are required to resolve these questions. Therefore, the main aims of the DenGate proposal are (1) to clarify the age of the first opening of the Denmark Strait by determining the ages of the oceanic basement and the oldest sediments and (2) to obtain a continuous record of the overflow water fluctuations across the Denmark Strait from contourite drift deposits near the gateway.
Multiple IODP, ODP, and DSDP sites in sediment drifts within the Norwegian Sea (north of the Greenland–Scotland Ridge) and the Rockall Trough and Iceland Basin (south of the Greenland–Scotland Ridge) are available to study the Paleogene–Holocene history of oceanic flow across the Faroe–Iceland Ridge (Fig. 5). This effort continued in 2023 with drilling of the Björn and Gardar drifts in the Iceland Basin by IODP Expedition 395, and seismic site surveying of sediment drifts in the Norwegian Sea is currently scheduled to support future drilling campaigns (Gabi Uenzelmann-Neben, personal communication, 2023). However, none of this activity can directly reconstruct DSOW. The complicating factor is that current branches diverge from the EGC close to the north of the Denmark Strait and converge with the EGC close to the south of the Denmark Strait (Fig. 5). Therefore, only by undertaking drilling close to the Denmark Strait itself can we directly reconstruct variations in DSOW and, thus, clarify the relative roles of the Denmark Strait and Faroe–Iceland Ridge gateways in controlling AMOC. The Snorri Drift is a contourite drift accumulating on the southern flank of the Denmark Strait ridge as well as beneath the Denmark Strait itself. Shallow cores in the Snorri Drift have been used to reconstruct the past 240 kyr of fluctuations in DSOW, but the drift has never been cored to basement (Andrews et al., 2021). There is very little information available on the sediments immediately north of the Denmark Strait, in the Blosseville Basin between Greenland and the Kolbeinsey Ridge. Therefore, the DenGate working group began by assembling and interpreting the very sparse legacy seismic data available from this region. A promising sediment drift target, which they name the Freydis Drift (Fig. 5), has been identified and appears to be thicker than the Snorri Drift (Jones et al., 2024). The next steps, which we aim to achieve during 2024, are to propose a seismic site survey of the Snorri and Freydis drifts and, simultaneously, to submit an outline proposal for drilling to the European Consortium for Ocean Research Drilling (ECORD).
The Greenland ice sheet is a tipping element within the Earth's climate system; thus, understanding its long-term stability is critical for anticipating future climate and sea level scenarios. Whereas scientific drilling has been implemented on the West Greenland margin and deep areas of the northern North Atlantic Ocean, Northeast Greenland constitutes a data gap preventing accurate reconstructions of the Cenozoic climate–tectonic evolution and hampering our ability to estimate ice sheet instability and associated sea level rise under the current trend of climate change. During the NorthGreen MagellanPlus workshop, knowledge gaps were highlighted, and scientific targets were addressed, with focus on developing a long-term research strategy for illuminating ice–ocean–tectonic interactions in Northeast Greenland. This includes further scientific drilling through IODP mission-specific platforms; currently, three drilling proposals are being developed with the aim of addressing pressing questions within the Ground-Truthing Future Climate Change flagship initiative.
No data sets were used in this article.
The following researchers participated in the NorthGreen MagellanPlus workshop: Allison Cluett (Buffalo University, New York, USA), Anne de Vernal (Université du Québec à Montréal, Montreal, Canada), Antonia Ruppel (BGR, Hanover, Germany), Bernard Coakley (University of Alaska, Anchorage, USA), Camilla Snowman Andresen (GEUS, Copenhagen, Denmark), Catalina Gebhardt (AWI, Bremerhaven, Germany), Christian Schiffer (Uppsala University, Uppsala, Sweden), Christian Tegner (Aarhus University, Aarhus, Denmark), Christoph Böttner (Kiel University, Kiel, Germany), Colm O'Cofaigh (Durham University, Durham, UK), Cornelia Spiegel-Behnke (University of Bremen, Bremen, Germany), David McInroy (BGS, Edinburgh, UK), Dave Roberts (Durham University, Durham, UK), Dieter Franke (BGR, Hanover, Germany), Drew Christ (University of Vermont, Burlington, USA), Eivind O. Straume (University of Texas at Austin, Austin, Texas, USA), Elizabeth Thomas (Buffalo University, New York, USA), Emilie R. Bennedsen (University of Copenhagen, Copenhagen Denmark), Gabriele Uenzelmann-Neben (AWI, Bremerhaven, Germany), Grace Shephard (University of Oslo, Oslo, Norway), Gregers Dam (GEUS, Copenhagen, Denmark), Gunnar Sand (SINTEF, Oslo, Norway), Henk Brinkhuis (Utrecht University, Utrecht, the Netherlands), James Barnett (Stockholm University, Stockholm, Sweden), Jason Briner (Buffalo University, New York, USA), Jeremy Lloyd (Durham University, Durham, UK), John Hopper (GEUS, Copenhagen, Denmark), Joseph Stoner (Oregon State University, Corvallis, USA), Jung-Hyun Kim (KOPRI, Incheon, South Korea), Karsten Gohl (AWI, Bremerhaven, Germany), Kasia Sliwinska (GEUS, Copenhagen, Denmark), Katrin Meier (University of Bremen, Bremen, Germany), Katrine Juul Andresen (Aarhus University, Aarhus, Denmark), Kelly Hogan (BAS, Cambridge, UK), Kwangchul Jang (KOPRI, Incheon, South Korea), Lara F. Pérez (GEUS, Aarhus, Denmark), Lis Allaart (GEUS, Aarhus, Denmark), Lutz Reinhardt (BGR, Hanover, Germany), Maciej Jez (Institute of Geological Sciences, Polish Academy of Sciences, Warsaw, Poland), Madeleine Larissa Vickers (University of Oslo, Oslo, Norway), Marit-Solveig Seidenkrantz (Aarhus University, Aarhus, Denmark), Martin Jakobsson (Stockholm University, Stockholm, Sweden), Mary-Lynn Dickson (RN Canada, Halifax, Canada), Matt O'Regan (Stockholm University, Stockholm, Sweden), Michael Bryld Wessel Fyhn (GEUS, Copenhagen, Denmark), Monica Winsborrow (UiT – The Arctic University of Norway, Tromsø Norway), Morten Bjerager (GEUS, Copenhagen, Denmark), Naima El bani Altuna (UiT – The Arctic University of Norway, Tromsø Norway), Niels Jákup Korsgaard (GEUS, Copenhagen, Denmark), Ole Bennike (GEUS, Copenhagen, Denmark), Paul Knutz (GEUS, Copenhagen, Denmark), Peter Klitzke (BGR, Hanover, Germany), Rebecca Pickering (Lund University, Lund, Sweden), Renata Giulia Lucchi (OGS, Trieste, Italy), Richard Levy (GNS Science, Wellington, New Zealand), Sarah Benetti (Ulster University, Ulster, Northern Ireland), Sean Gulick (Texas A&M, College Station, USA), Seung-Il Nam (KOPRI, Incheon, South Korea), Sofia Ribeiro (GEUS, Copenhagen, Denmark), Stephen Grasby (Geological Survey of Canada, Alberta, Canada), Stephen Jones (University of Birmingham, Birmingham, UK), Sverre Planke (VBPR, Oslo, Norway), Thomas Funk (GEUS, Copenhagen, Denmark), Thorsten Nagel (Aarhus University, Aarhus, Denmark), Tove Nielsen (GEUS, Copenhagen, Denmark), Trine Dahl-Jensen (GEUS, Copenhagen, Denmark), Vivi Kathrine Pedersen (Aarhus University, Aarhus, Denmark), Wei-Li Hong (Stockholm University, Stockholm, Sweden), and Wolfram Geissler (AWI, Bremerhaven, Germany).
LFP wrote the core portion of the manuscript and was the main organizer of the NorthGreen MagellanPlus workshop. LFP led the 1011-Pre proposal. PCK, JRH, MSS, and MO'R provided input on the manuscript text and were co-organizers of the workshop. MSS was the ECORD-appointed watchdog during workshop organization. JRH made the figure base maps. As proposal leader, MO'R wrote the core portion of Sect. 4.2.1. As leader of DenGate initiative, SJ provided input on the manuscript text and wrote the core portion of Sect. 4.2.3.
The contact author has declared that none of the authors has any competing interests.
Publisher’s note: Copernicus Publications remains neutral with regard to jurisdictional claims made in the text, published maps, institutional affiliations, or any other geographical representation in this paper. While Copernicus Publications makes every effort to include appropriate place names, the final responsibility lies with the authors.
Funding for the organization of the NorthGreen MagellanPlus workshop was provided by the MagellanPlus Workshop Series Programme of ECORD and by the Carlsberg Foundation. We acknowledge the participation and enthusiasm of all of the workshop participants. We acknowledge the editor Nadine Hallmann and the reviews by Wilfried Jokat and an anonymous reviewer which greatly improved the initial version of this work.
This research has been supported by the Carlsbergfondet (grant no. CF22-0160) and the MagellanPlus Workshop Series Programme of ECORD.
This paper was edited by Nadine Hallmann and reviewed by Wilfried Jokat and one anonymous referee.
Abdelmalak, M. M., Gac, S., Faleide, J. I., Shephard, G. E., Tsikalas, F., Polteau, S., Zastrozhnov, D., and Torsvik, T. H.: Quantification and Restoration of the Pre-Drift Extension Across the NE Atlantic Conjugate Margins During the Mid-Permian-Early Cenozoic Multi-Rifting Phases, Tectonics, 42, e2022TC007386, https://doi.org/10.1029/2022tc007386, 2023.
Andrews, J. T., McCave, I. N., and Syvitski, J.: A ∼ 240 ka record of Ice Sheet and Ocean interactions on the Snorri Drift, SW of Iceland, Global Planet. Change, 201, 103498, https://doi.org/10.1016/j.gloplacha.2021.103498, 2021.
Arndt, J. E., Jokat, W., Dorschel, B., Myklebust, R., Dowdeswell, J. A., and Evans, J.: A new bathymetry of the Northeast Greenland continental shelf: Constraints on glacial and other processes, Geochem. Geophy. Geosy., 16, 3733–3753, https://doi.org/10.1002/2015GC005931, 2015.
Bailey, I., Hole, G. M., Foster, G. L., Wilson, P. A., Storey, C. D., Trueman, C. N., and Raymo, M. E.: An alternative suggestion for the Pliocene onset of major northern hemisphere glaciation based on the geochemical provenance of North Atlantic Ocean ice-rafted debris, Quaternary Sci. Rev., 75, 181–194, https://doi.org/10.1016/j.quascirev.2013.06.004, 2013.
Bennike, O., Abrahamsen, N., Bak, M., Israelson, C., Konradi, P., Matthiessen, J., and Witkowski, A.: A multi-proxy study of Pliocene sediments from Île de France, North-East Greenland, Palaeogeogr. Palaeocl., 186, 1–23, https://doi.org/10.1016/S0031-0182(02)00439-X, 2002.
Bennike, O. L. E., Knudsen, K. L., Abrahamsen, N., BÖCher, J., Cremer, H., and Wagner, B.: Early Pleistocene sediments on Store Koldewey, northeast Greenland, Boreas, 39, 603–619, https://doi.org/10.1111/j.1502-3885.2010.00147.x, 2010.
Berger, D. and Jokat, W.: A seismic study along the East Greenland margin from 72° N to 77° N, Geophys. J. Int., 174, 733–748, https://doi.org/10.1111/j.1365-246X.2008.03794.x, 2008.
Berger, D. and Jokat, W.: Sediment deposition in the northern basins of the North Atlantic and characteristic variations in shelf sedimentation along the East Greenland Margin, Mar. Petrol. Geol., 26, 1321–1337, https://doi.org/10.1016/j.marpetgeo.2009.04.005, 2009.
Bierman, P. R., Shakun, J. D., Corbett, L. B., Zimmerman, S. R., and Rood, D. H.: A persistent and dynamic East Greenland ice sheet over the past 7.5 million years, Nature, 540, 256–260, https://doi.org/10.1038/nature20147, 2016.
Böning, C. W., Behrens, E., Biastoch, A., Getzlaff, K., and Bamber, J. L.: Emerging impact of Greenland meltwater on deepwater formation in the North Atlantic Ocean, Nat. Geosci., 9, 523–527, https://doi.org/10.1038/ngeo2740, 2016.
Brozena, J. M., Childers, V. A., Lawver, L. A., Gahagan, L. M., Forsberg, R., Faleide, J. I., and Eldholm, O.: New aerogeophysical study of the Eurasia Basin and Lomonosov Ridge: Implications for basin development, Geology, 31, 825–828, https://doi.org/10.1130/G19528.1, 2003.
Budéus, G. and Schneider, W.: On the hydrography of the Northeast Water Polynya, J. Geophys. Res., 100, 4287–4299, https://doi.org/10.1029/94JC02024, 1995.
Caesar, L., McCarthy, G. D., Thornalley, D. J. R., Cahill, N., and Rahmstorf, S.: Current Atlantic Meridional Overturning Circulation weakest in last millennium, Nat. Geosci., 14, 118–120, https://doi.org/10.1038/s41561-021-00699-z, 2021.
Christ, A. J., Rittenour, T. M., Bierman, P. R., Keisling, B. A., Knutz, P. C., Thomsen, T. B., Keulen, N., Fosdick, J. C., Hemming, S. R., Tison, J.-L., Blard, P.-H., Steffensen, J. P., Caffee, M. W., Corbett, L. B., Dahl-Jensen, D., Dethier, D. P., Hidy, A. J., Perdrial, N., Peteet, D. M., Steig, E. J., and Thomas, E. K.: Deglaciation of northwestern Greenland during Marine Isotope Stage 11, Science, 381, 330–335, https://doi.org/10.1126/science.ade4248, 2023.
Christiansen, F. G.: Greenland petroleum exploration history: Rise and fall, learnings, and future perspectives, Resour. Policy, 74, 102425, https://doi.org/10.1016/j.resourpol.2021.102425, 2021.
Clotten, C., Stein, R., Fahl, K., Schreck, M., Risebrobakken, B., and De Schepper, S.: On the causes of Arctic sea ice in the warm Early Pliocene, Sci. Rep., 9, 989, https://doi.org/10.1038/s41598-018-37047-y, 2019.
Damm, E. A.: The Expedition PS115/1 of the Research Vessel POLARSTERN to the Greenland Sea and Wandel Sea in 2018, Alfred Wegener Institute for Polar and Marine Research, Bremerhaven, https://doi.org/10.2312/BzPM_0727_2019, 2019.
Darby, D. A.: Ephemeral formation of perennial sea ice in the Arctic Ocean during the middle Eocene, Nat. Geosci., 7, 210–213, https://doi.org/10.1038/ngeo2068, 2014.
Davies, S., Stow, D., and Nicholson, U.: Late glacial to Holocene sedimentary facies of the Eirik Drift, southern Greenland margin: Spatial and temporal variability and paleoceanographic implications, Mar. Geol., 440, 106568, https://doi.org/10.1016/j.margeo.2021.106568, 2021.
De Schepper, S., Schreck, M., Beck, K. M., Matthiessen, J., Fahl, K., and Mangerud, G.: Early Pliocene onset of modern Nordic Seas circulation related to ocean gateway changes, Nat. Commun., 6, 8659, https://doi.org/10.1038/ncomms9659, 2015.
Døssing, A., Japsen, P., Watts, A. B., Nielsen, T., Jokat, W., Thybo, H., and Dahl-Jensen, T.: Miocene uplift of the NE Greenland margin linked to plate tectonics: Seismic evidence from the Greenland Fracture Zone, NE Atlantic, Tectonics, 35, 257–282, https://doi.org/10.1002/2015TC004079, 2016.
Dutton, A., Carlson, A. E., Long, A. J., Milne, G. A., Clark, P. U., DeConto, R., Horton, B. P., Rahmstorf, S., and Raymo, M. E.: Sea-level rise due to polar ice-sheet mass loss during past warm periods, Science, 349, aaa4019, https://doi.org/10.1126/science.aaa4019, 2015.
Ehlers, B.-M. and Jokat, W.: Paleo-bathymetry of the northern North Atlantic and consequences for the opening of the Fram Strait, Mar. Geophys. Res., 34, 25–43, https://doi.org/10.1007/s11001-013-9165-9, 2013.
Engen, Ø., Faleide, J. I., and Dyreng, T. K.: Opening of the Fram Strait gateway: A review of plate tectonic constraints, Tectonophysics, 450, 51–69, https://doi.org/10.1016/j.tecto.2008.01.002, 2008.
Escutia, C., DeConto, R., Dunbar, R., De Santis, L., Shevenell, A., and Nash, T.: Keeping an Eye on Antarctic Ice Sheet Stability, Oceanography, 32, 32–46, https://doi.org/10.5670/oceanog.2019.117, 2019.
Fahnestock, M., Abdalati, W., Joughin, I., Brozena, J., and Gogineni, P.: High Geothermal Heat Flow, Basal Melt, and the Origin of Rapid Ice Flow in Central Greenland, Science, 294, 2338–2342, https://doi.org/10.1126/science.1065370, 2001.
Fyhn, M. B. W. and Hopper, J. R.: NE Greenland Composite Tectono-Sedimentary Element, northern Greenland Sea and Fram Strait, Geological Society, London, Memoirs, 57, M57-2017-2012, https://doi.org/10.1144/M57-2017-12, 2024.
Gaina, C., Nasuti, A., Kimbell, G. S., and Blischke, A.: Break-up and seafloor spreading domains in the NE Atlantic, in: The NE Atlantic Region: A Reappraisal of Crustal Structure, Tectonostratigraphy and Magmatic Evolution, edited by: Pinvidic, G., Hopper, J. R., Stoker, M. S., Gaina, C., Doornenbal, J. C., Funck, T., and Árting, U. E., Geological Society of London, 393–417, https://doi.org/10.1144/sp447.12, 2017.
Gion, A. M., Williams, S. E., and Müller, R. D.: A reconstruction of the Eurekan Orogeny incorporating deformation constraints, Tectonics, 36, 304–320, https://doi.org/10.1002/2015tc004094, 2017.
Golledge, N. R., Keller, E. D., Gomez, N., Naughten, K. A., Bernales, J., Trusel, L. D., and Edwards, T. L.: Global environmental consequences of twenty-first-century ice-sheet melt, Nature, 566, 65–72, https://doi.org/10.1038/s41586-019-0889-9, 2019.
GreenDrill: https://greendrill-cosmo.ldeo.columbia.edu/, last access: June 2023.
Hamann, N. E., Whittaker, R. C., and Stemmerik, L.: Geological development of the Northeast Greenland shelf, in: Petroleum Geology: North-West Europe and Global Perspectives – Proceedings of the 6th Petroleum Geology Conference, edited by: Doré, A. G. and Vining, B. A., Petroleum Geology Conferences Ltd., Geological Society, London, 887–902, 2005.
Hansen, B., Østerhus, S., Quadfasel, D., and Turrell, B.: Already the Day After Tomorrow?, Science, 305, 953–954, https://doi.org/10.1126/science.1100085, 2004.
Hardarson, B. S., Fitton, J. G., Ellam, R. M., and Pringle, M. S.: Rift relocation – A geochemical and geochronological investigation of a palaeo-rift in northwest Iceland, Earth Planet. Sc. Lett., 153, 181–196, https://doi.org/10.1016/S0012-821X(97)00145-3, 1997.
Heirman, K., Nielsen, T., and Kuijpers, A.: Impact of Tectonic, Glacial and Contour Current Processes on the Late Cenozoic Sedimentary Development of the Southeast Greenland Margin, Geosciences, 9, 157, https://doi.org/10.3390/geosciences9040157, 2019.
Hofer, S., Lang, C., Amory, C., Kittel, C., Delhasse, A., Tedstone, A., and Fettweis, X.: Greater Greenland ice sheet contribution to global sea level rise in CMIP6, Nat. Commun., 11, 6289, https://doi.org/10.1038/s41467-020-20011-8, 2020.
IPCC: Summary for Policymakers, in: IPCC Special Report on the Ocean and Cryosphere in a Changing Climate, edited by: Pörtner, H. O., Roberts, D. C., Masson-Delmotte, V., Zhai, P., Tignor, M., Poloczanska, E., Mintenbeck, K., Alegría, A., Nicolai, M., Okem, A., Petzold, J., Rama, B., and Weyer, N. M., in press, 2019.
Jakobsson, M., Backman, J., Rudels, B., Nycander, J., Frank, M., Mayer, L., Jokat, W., Sangiorgi, F., O'Regan, M., Brinkhuis, H., King, J., and Moran, K.: The early Miocene onset of a ventilated circulation regime in the Arctic Ocean, Nature, 447, 986–990, https://doi.org/10.1038/nature05924, 2007.
Jakobsson, M., Mayer, L., Coakley, B., Dowdeswell, J. A., Forbes, S., Fridman, B., Hodnesdal, H., Noormets, R., Pedersen, R., Rebesco, M., Schenke, H. W., Zarayskaya, Y., Accettella, D., Armstrong, A., Anderson, R. M., Bienhoff, P., Camerlenghi, A., Church, I., Edwards, M., Gardner, J. V., Hall, J. K., Hell, B., Hestvik, O., Kristoffersen, Y., Marcussen, C., Mohammad, R., Mosher, D., Nghiem, S. V., Pedrosa, M. T., Travaglini, P. G., and Weatherall, P.: The International Bathymetric Chart of the Arctic Ocean (IBCAO) Version 3.0, Geophys. Res. Lett., 39, L12609, https://doi.org/10.1029/2012GL052219, 2012.
Jansen, E., Raymo, M. E., and Blum, P.: Proceedings, initial reports, Ocean Drilling Program, Leg 162, North Atlantic-Arctic gateways II, ODP, Texas A and M University, College Station, ISSN 0884-5883, 1996.
Jokat, W., Lehmann, P., Damaske, D., and Bradley Nelson, J.: Magnetic signature of North-East Greenland, the Morris Jesup Rise, the Yermak Plateau, the central Fram Strait: Constraints for the rift/drift history between Greenland and Svalbard since the Eocene, Tectonophysics, 12, 98–109, https://doi.org/10.1016/j.tecto.2015.12.002, 2015.
Jones, S. M., Hopper, J. R., Pérez, L. F., Hall, J. R., Uenzelmann-Neben, G., and Fyhn, M.: Potential for scientific drilling of sediment drifts adjacent to Denmark Strait oceanic gateway, Geological Society of London Special Publication, in review, 2024.
Knutz, P. C., Jennings, A., Childress, L. B., and Scientists, T. E.: Expedition 400 Preliminary Report: NW Greenland Glaciated Margin, International Ocean Discovery Program Preliminary Report, International Ocean Discovery Program, https://doi.org/10.14379/iodp.pr.400.2024, 2024.
Koppers, A. and Coggon, R.: Exploring Earth by scientific ocean drilling, 2050 Science Framework, 124, 2020.
Kristoffersen, Y., Tholfsen, A., Hall, J. K., and Stein, R.: Scientists spend Arctic winter adrift on sea ice, Eos, 97, 1/10, https://doi.org/10.1029/2016EO060711, 2016.
Kristoffersen, Y., Hall, J. K., and Nilsen, E. H.: Morris Jesup Spur and Rise north of Greenland – exploring present seabed features, the history of sediment deposition, volcanism and tectonic deformation at a Late Cretaceous/early Cenozoic triple junction in the Arctic Ocean, Norw. J. Geol., 101, 202104, https://doi.org/10.17850/njg101-1-4, 2021.
Larsen, H. C., Saunders, A. D., Clift, P. D., Ali, J., Begét, J., Cambray, H., Demant, A., Fitton, G., Fram, M. S., Fukuma, K., Gieskes, J., Holmes, M. A., Hunt, J., Lacasse, C., Larsen, L. M., Lykke-Anderson, H., Meltser, A., Morrison, M. L., Nemoto, N., Okay, N., Saito, S., Sinton, C., Spezzaferri, S., Stax, R., Vallier, T. L., Vandamme, D., Wei, W., and Werner, R.: Seven million years of glaciation in Greenland, Science, 264, 952–955, 1994.
Larsen, N. K., Levy, L. B., Carlson, A. E., Buizert, C., Olsen, J., Strunk, A., Bjørk, A. A., and Skov, D. S.: Instability of the Northeast Greenland Ice Stream over the last 45,000 years, Nat. Commun., 9, 1872–1872, https://doi.org/10.1038/s41467-018-04312-7, 2018.
Lucchi, R. G., St. John, K., and Ronge, T. A.: Expedition 403 Scientific Prospectus: Eastern Fram Strait Paleo-Archive (FRAME), International Ocean Discovery Program Scientific Prospectus, IODP, https://doi.org/10.14379/iodp.sp.403.2023, 2023.
Maslin, M. A., Li, X. S., Loutre, M. F., and Berger, A.: The contribution of orbital forcing to the progressive intensification of Northern Hemisphere Glaciation, Quaternary Sci. Rev., 17, 411–426, https://doi.org/10.1016/S0277-3791(97)00047-4, 1998.
Morlighem, M., Williams, C. N., Rignot, E., An, L., Arndt, J. E., Bamber, J. L., Catania, G., Chauché, N., Dowdeswell, J. A., Dorschel, B., Fenty, I., Hogan, K., Howat, I., Hubbard, A., Jakobsson, M., Jordan, T. M., Kjeldsen, K. K., Millan, R., Mayer, L., Mouginot, J., Noël, B. P. Y., O'Cofaigh, C., Palmer, S., Rysgaard, S., Seroussi, H., Siegert, M. J., Slabon, P., Straneo, F., van den Broeke, M. R., Weinrebe, W., Wood, M., and Zinglersen, K. B.: BedMachine v3: Complete Bed Topography and Ocean Bathymetry Mapping of Greenland From Multibeam Echo Sounding Combined With Mass Conservation, Geophys. Res. Lett., 44, 11051–11061, https://doi.org/10.1002/2017gl074954, 2017.
Newton, R., Pfirman, S., Tremblay, L. B., and DeRepentigny, P.: Defining the “Ice Shed” of the Arctic Ocean's Last Ice Area and Its Future Evolution, Earths Future, 9, e2021EF001988, https://doi.org/10.1029/2021ef001988, 2021.
Notz, D. and SIMIP Community: Arctic Sea Ice in CMIP6, Geophys. Res. Lett., 47, e2019GL086749, https://doi.org/10.1029/2019gl086749, 2020.
Parnell-Turner, R., White, N. J., McCave, I. N., Henstock, T. J., Murton, B., and Jones, S. M.: Architecture of North Atlantic contourite drifts modified by transient circulation of the Icelandic mantle plume, Geochem. Geophy. Geosy., 16, 3414–3435, https://doi.org/10.1002/2015GC005947, 2015.
Pérez, L. F., Nielsen, T., Knutz, P. C., Kuijpers, A., and Damm, V.: Large-scale evolution of the central-east Greenland margin: New insights to the North Atlantic glaciation history, Global Planet. Change, 163, 141–157, https://doi.org/10.1016/j.gloplacha.2017.12.010, 2018.
Poirier, A. and Hillaire-Marcel, C.: Improved Os-isotope stratigraphy of the Arctic Ocean, Geophys. Res. Lett., 38, L14607, https://doi.org/10.1029/2011gl047953, 2011.
Poore, H. R., Samworth, R., White, N. J., Jones, S. M., and McCave, I. N.: Neogene overflow of Northern Component Water at the Greenland-Scotland Ridge, Geochem. Geophy. Geosy., 7, Q06010, https://doi.org/10.1029/2005gc001085, 2006.
Rahmstorf, S.: Ocean circulation and climate during the past 120,000 years, Nature, 419, 207–214, https://doi.org/10.1038/nature01090, 2002.
Rignot, E., Velicogna, I., van den Broeke, M. R., Monaghan, A., and Lenaerts, J. T. M.: Acceleration of the contribution of the Greenland and Antarctic ice sheets to sea level rise, Geophys. Res. Lett., 38, L05503, https://doi.org/10.1029/2011gl046583, 2011.
Rudels, B.: Arctic Ocean Circulation, in: Encyclopedia of Ocean Sciences, Elsevier Ltd, 211–225, https://doi.org/10.1016/B978-012374473-9.00601-9, 2009.
Schaefer, J. M., Finkel, R. C., Balco, G., Alley, R. B., Caffee, M. W., Briner, J. P., Young, N. E., Gow, A. J., and Schwartz, R.: Greenland was nearly ice-free for extended periods during the Pleistocene, Nature, 540, 252–255, https://doi.org/10.1038/nature20146, 2016.
Schaffer, J., Kanzow, T., von Appen, W.-J., von Albedyll, L., Arndt, J. E., and Roberts, D. H.: Bathymetry constrains ocean heat supply to Greenland's largest glacier tongue, Nat. Geosci., 13, 227–231, https://doi.org/10.1038/s41561-019-0529-x, 2020.
Shepherd, A., Ivins, E., Rignot, E., Smith, B., van den Broeke, M., Velicogna, I., Whitehouse, P., Briggs, K., Joughin, I., Krinner, G., Nowicki, S., Payne, T., Scambos, T., Schlegel, N., A, G., Agosta, C., Ahlstrøm, A., Babonis, G., Barletta, V. R., Bjørk, A. A., Blazquez, A., Bonin, J., Colgan, W., Csatho, B., Cullather, R., Engdahl, M. E., Felikson, D., Fettweis, X., Forsberg, R., Hogg, A. E., Gallee, H., Gardner, A., Gilbert, L., Gourmelen, N., Groh, A., Gunter, B., Hanna, E., Harig, C., Helm, V., Horvath, A., Horwath, M., Khan, S., Kjeldsen, K. K., Konrad, H., Langen, P. L., Lecavalier, B., Loomis, B., Luthcke, S., McMillan, M., Melini, D., Mernild, S., Mohajerani, Y., Moore, P., Mottram, R., Mouginot, J., Moyano, G., Muir, A., Nagler, T., Nield, G., Nilsson, J., Noël, B., Otosaka, I., Pattle, M. E., Peltier, W. R., Pie, N., Rietbroek, R., Rott, H., Sandberg Sørensen, L., Sasgen, I., Save, H., Scheuchl, B., Schrama, E., Schröder, L., Seo, K.-W., Simonsen, S. B., Slater, T., Spada, G., Sutterley, T., Talpe, M., Tarasov, L., van de Berg, W. J., van der Wal, W., van Wessem, M., Vishwakarma, B. D., Wiese, D., Wilton, D., Wagner, T., Wouters, B., Wuite, J., and The IMBIE Team: Mass balance of the Greenland Ice Sheet from 1992 to 2018, Nature, 579, 233–239, https://doi.org/10.1038/s41586-019-1855-2, 2020.
Smith, A. G. and Pickering, K. T.: Oceanic gateways as a critical factor to initiate icehouse Earth, J. Geol. Soc., 160, 337–340, https://doi.org/10.1144/0016-764902-115, 2003.
Solheim, A., Faleide, J. I., Andersen, E. S., Elverhøi, A., Forsberg, C. F., Vanneste, K., Uenzelmann-Neben, G., and Channell, J. E. T.: Late cenozoic seismic stratigraphy and glacial geological development of the East Greenland and Svalbard-Barents sea continental margins, Quaternary Sci. Rev., 17, 155–184, https://doi.org/10.1016/S0277-3791(97)00068-1, 1998.
St. John, K. E. K. and Krissek, L. A.: The late Miocene to Pleistocene ice-rafting history of Southeast Greenland, Boreas, 31, 28–35, https://doi.org/10.1111/j.1502-3885.2002.tb01053.x, 2002.
Stoker, M. S., Stewart, M. A., Shannon, P. M., Bjerager, M., Nielsen, T., Blischke, A., Hjelstuen, B. O., Gaina, C., McDermott, K., and Ólavsdóttir, J.: An overview of the Upper Palaeozoic–Mesozoic stratigraphy of the NE Atlantic region, Geol. Soc. Spec. Publ., 447, 11–68, https://doi.org/10.1144/sp447.2, 2016.
Straneo, F., Sutherland, D. A., Holland, D., Gladish, C., Hamilton, G. S., Johnson, H. L., Rignot, E., Xu, Y., and Koppes, M.: Characteristics of ocean waters reaching Greenland's glaciers, Ann. Glaciol., 53, 202–210, https://doi.org/10.3189/2012AoG60A059, 2017.
Straume, E. O., Gaina, C., Medvedev, S., and Nisancioglu, K. H.: Global Cenozoic Paleobathymetry with a focus on the Northern Hemisphere Oceanic Gateways, Gondwana Res., 86, 126–143, https://doi.org/10.1016/j.gr.2020.05.011, 2020.
Talwani, M. and Eldholm, O.: Evolution of the Norwegian-Greenland Sea, Geol. Soc. Am. Bull., 88, 969–999, https://doi.org/10.1130/0016-7606(1977)88<969:EOTNS>2.0.CO;2, 1977.
Thiede, J., Myhre, A. M., Firth, J. V., Johnson, G. L., and Ruddiman, W. F.: Procedings ODP Scientific Results Ocean Drilling Program, College Station, 1996.
Thiede, J., Jessen, C., Knutz, P., Kuijpers, A., Mikkelsen, N., Nørgaard-Pedersen, N., and Spielhagen, R. F.: Millions of years of Greenland ice sheet history recorded in Ocean sediments, Polarforschung, 80, 141–159, http://hdl.handle.net/10013/epic.38391.d001 (last access: June 2023), 2010.
Tripati, A. K., Eagle, R. A., Morton, A., Dowdeswell, J. A., Atkinson, K. L., Bahé, Y., Dawber, C. F., Khadun, E., Shaw, R. M. H., Shorttle, O., and Thanabalasundaram, L.: Evidence for glaciation in the Northern Hemisphere back to 44 Ma from ice-rafted debris in the Greenland Sea, Earth Planet. Sc. Lett., 265, 112–122, https://doi.org/10.1016/j.epsl.2007.09.045, 2008.
Tsikalas, F., Faleide, J. I., Eldholm, O., and Antonio Blaich, O.: The NE Atlantic conjugate margins, in Regional Geology and Tectonics: Phanerozoic Passive Margins, Cratonic Basins and Global Tectonic Maps, Elsevier, 140–201, https://doi.org/10.1016/b978-0-444-56357-6.00004-4, 2012.
Våge, K., Pickart, R. S., Spall, M. A., Moore, G. W. K., Valdimarsson, H., Torres, D. J., Erofeeva, S. Y., and Nilsen, J. E. Ø.: Revised circulation scheme north of the Denmark Strait, Deep-Sea Res. Pt. I, 79, 20–39, https://doi.org/10.1016/j.dsr.2013.05.007, 2013.
van den Broeke, M. R., Enderlin, E. M., Howat, I. M., Kuipers Munneke, P., Noël, B. P. Y., van de Berg, W. J., van Meijgaard, E., and Wouters, B.: On the recent contribution of the Greenland ice sheet to sea level change, The Cryosphere, 10, 1933–1946, https://doi.org/10.5194/tc-10-1933-2016, 2016.
White, R. S., Bown, J. W., and Smallwood, J. R.: The temperature of the Iceland plume and origin of outward-propagating V-shaped ridges, J. Geol. Soc., 152, 1039–1045, https://doi.org/10.1144/gsl.jgs.1995.152.01.26, 1995.
Wolf, T. C. W. and Thiede, J.: History of terrigenous sedimentation during the past 10 m.y. in the North Atlantic (ODP Legs 104 and 105 and DSDP Leg 81), Mar. Geol., 101, 83–102, https://doi.org/10.1016/0025-3227(91)90064-B, 1991.
Wright, J. D. and Miller, K. G.: Control of North Atlantic Deep Water circulation by the Greenland-Scotland Ridge, Paleocoanography, 11, 157–170, 1996.
Zwally, H. J., Giovinetto, M. B., Beckley, M. A., and Saba, J. L.: Antarctic and Greenland Drainage Systems, GSFC Cryospheric Sciences Laboratory, https://earth.gsfc.nasa.gov/cryo/data/polar-altimetry/antarctic-and-greenland-drainage-systems (last access: June 2023), 2012.
- Abstract
- Introduction
- Scientific background: tectonics, oceanography, and cryosphere in Northeast Greenland
- Workshop scope and objectives
- Workshop structure and outcomes
- Concluding remarks and future work
- Data availability
- Workshop participants list
- Author contributions
- Competing interests
- Disclaimer
- Acknowledgements
- Financial support
- Review statement
- References
- Abstract
- Introduction
- Scientific background: tectonics, oceanography, and cryosphere in Northeast Greenland
- Workshop scope and objectives
- Workshop structure and outcomes
- Concluding remarks and future work
- Data availability
- Workshop participants list
- Author contributions
- Competing interests
- Disclaimer
- Acknowledgements
- Financial support
- Review statement
- References