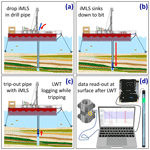
New geophysical memory-logging system for highly unstable and inclined scientific exploration drilling
Marco Groh
Martin Töpfer
Andreas Jurczyk
Ulrich Harms
We established a cable-free memory-logging system for drill-string-deployed geophysical borehole measurements. For more than 20 years, various so-called “logging while tripping” (LWT) techniques have been available in the logging service industry. However, this method has rarely been used in scientific drilling, although it enables logging in deviated and unstable boreholes, such as in lacustrine sediment drilling projects. LWT operations have a far lower risk of damage or loss of downhole logging equipment compared with the common wireline logging. For this purpose, we developed, tested, and commissioned a modular memory-logging system that does not require drill string modifications, such as special collars, and can be deployed in standard wireline core drilling diameters (HQ, bit size of 96 mm, and PQ, bit size of 123 mm). The battery-powered, autonomous sondes register the profiles of the natural GR (gamma radiation) spectrum, sonic velocity, magnetic susceptibility, electric resistivity, temperature, and borehole inclination in high quality while they are pulled out along with the drill string. As a precise depth measurement carried out in the drill rig is just as important as the actual petrophysical downhole measurements, we developed depth-measuring devices providing a high accuracy of less than 0.1 m deviation from the wireline-determined depth. Moreover, the modular structure of the system facilitates sonde deployment in online mode for wireline measurements.
- Article
(9720 KB) - Full-text XML
- BibTeX
- EndNote
Borehole measurements are an indispensable part of scientific drilling projects to gain physical and chemical parameters continuously and in situ (Goldberg, 1997). They are usually carried out using the well-established wireline logging method, in which the measuring sondes are moved on a logging cable in the borehole. This method has the advantages of power supply to the sondes, online data transfer to the recording unit at surface, and very precise and reliable depth measurements. Furthermore, it is easy and flexible to use, comprises the largest range of sonde types, and is readily available through a large number of academic and commercial providers. In the framework of the International Continental Scientific Drilling Program (ICDP), we have conducted more than 37 wireline logging campaigns (Hodell et al., 2006; Koeberl et al., 2007; Gebhardt et al., 2013; Jackson et al., 2019; Jerram et al., 2019; Abbott and Rodbell, 2020) using slimhole tools. For the majority of these missions, most demands could be covered. However, there is also a significant proportion of projects in which unintentional or technically necessary downhole conditions adverse to wireline logging occur, making the use of logging sondes on a cable risky, extremely difficult, and, therefore, very time-consuming or even impossible. The most common adversities are partially blocked boreholes (e.g., large-scale wall collapse or local bridging) and borehole paths that deviate strongly from vertical.
In lacustrine sediment coring projects, operations are performed without casing; thus, unconsolidated sediments occurring over long sections often rapidly narrow boreholes and make them impassable for lightweight logging sondes. Mostly, however, a machine-powered drill string can pass through without problems or is used to redrill the well quickly. In such cases, the usual wireline logging procedure is to position the drill string at a depth with the drill bit below the suspected unstable section and to run the wireline sondes through the string into the open hole section below the bit. After measuring this section with all desired sondes, the drill string is pulled higher, leaving the unstable section uncased. These steps are repeated as often as the formation instabilities require (Baumgarten and Wonik, 2015). Overall, this is a very time-consuming procedure and can take up to 10 times longer than normal, unimpeded wireline logging in a several-hundred-meter-deep hole. Another obstacle is that wireline sondes are always run through the drill bit into the open hole. This causes a high risk of getting stuck at the drill bit when reentering.
These limitations of wireline logging are currently hampering scientific and commercial operations; hence, there is a need for the invention of novel methods to perform downhole logging, especially under adverse conditions. The most prominent technique is the integration of logging sensors into the drill string (logging while drilling, LWD), which is widely used in complex hydrocarbon exploration (Hansen and White, 1991) and in scientific ocean drilling (e.g., Moore, 2000). Other deployment options include pipe-conveyed logging or stiff wireline logging, but these techniques are mostly associated with significantly higher costs compared with wireline logging. Another method has also frequently been used in scientific drilling involving robust wireless memory sondes that are mounted atop the inner core barrel, typically delivering parameters such as temperature, pressure, and acceleration, among others, which can be measured from inside a drill pipe (Guerin and Goldberg, 2002).
For the logging of geophysical parameters, one cost-effective and minimum-risk method for drilling projects is the application of so-called memory-logging sondes (Singh et al., 2018). These sondes are not run on a logging cable, but measure autonomously at the end of the drill string after they have been dropped into the drill string (very much like an inner core barrel), and land on the landing shoulder in the outer core barrel at the drill bit. When the drill string is pulled out of the borehole (trip-out), the sondes are pulled along with it and the data are stored in the sonde, which is why this method is commonly referred to as “logging while tripping” (LWT; Figs. 1, 2; (Matheson and West, 2000).
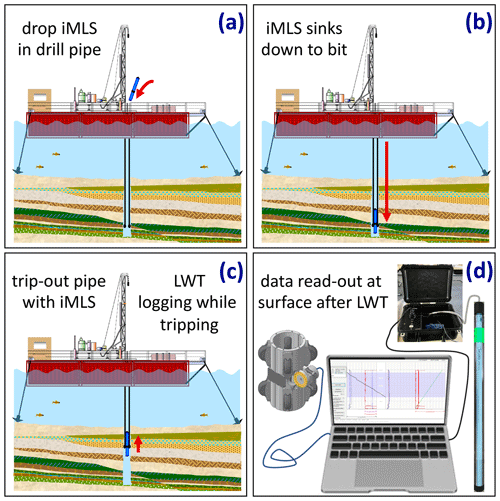
Figure 1Representation of the logging while tripping (LWT) method in a lake coring setup: (a) dropping the ICDP memory-logging system (iMLS) in the drill string, (b) landing the iMLS in the outer core barrel at the drill bit, (c) trip-out of the drill string and alongside logging with the iMLS, and (d) reading out the data from the iMLS sonde memory and the iMLS depth-measuring device (iDMD).
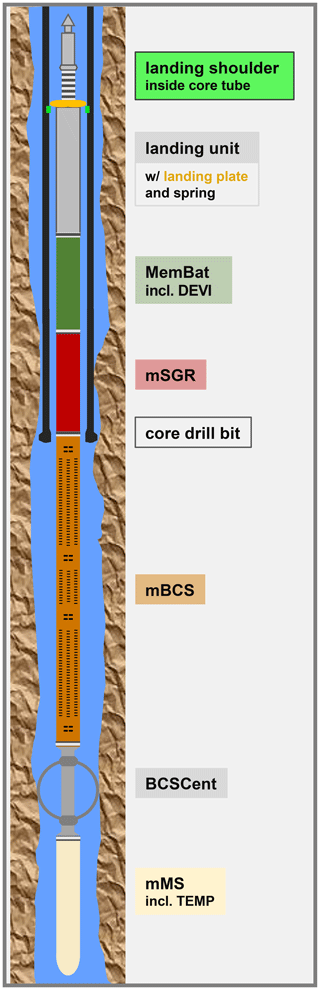
Figure 2Sketch of a standard ICDP memory-logging system (iMLS) tool combination landed at the bottom of the drill string, partially sticking out into the open hole in a ready-to-log position (not to scale).
The depth of each measuring point is determined at the surface as in wireline logging, but the assignment of the depth value to the measured value does not simultaneously take place during the measuring process; rather, the depth value is assigned to the measured value after the sonde data have been read out back on the surface. The combination of each individual downhole measured value with the associated surface depth value takes place by means of the measurement time (time stamp) registered in parallel at surface and down the hole. In other words, a downhole value with the same time as a depth value is assigned to this depth value. The depth and the downhole values are both deleted for times when the drill string is not moved upward, resulting a continuous, evenly decreasing depth profile of the downhole parameter. The LWT depth measurement in the drill rig is similar to the LWD depth determination; however, in the latter, the entire running-in of the drill string is measured from its very beginning at the derrick floor. LWT depth measurements can only begin when the last core has been drilled and brought to surface, i.e., the drill string has already been in the borehole for many core runs.
For LWT systems, the risks described above for wireline sondes are virtually negligible when running through unstable zones, because the tools only partially stick out of the core bit into the open hole. Moreover, the upper part of the tools always remains secure inside the drill string, which, on the one hand, completely eliminates the reentry risk and, on the other hand, minimizes exposure to the unstable hole. Should the LWT nevertheless get stuck, drillers can circulate drill mud through the drill bit in order to remove the blockage, and the tools in the drill string can easily be pulled with the high force of the drill rig. Under regular working conditions, the tool string can be retrieved at any time using the core retrieval device (coring wire line and overshot) – for example, if only a partial section of the borehole is to be logged or if technical drilling problems should arise.
Different variants of the logging while tripping method are used commercially worldwide (e.g., Aivalis et al., 2012; Beal, 2019). In the most widespread method, a special modified drill collar above the drill bit allows for the logging of some parameters from entirely within the pipe so that the logging tools never run into the open hole. Since 2012, a set of specially designed memory sondes have been deployed with the MeBo ocean floor drilling robot (Freudenthal et al., 2020), with most of the logging tools actually protruding out of the drill bit into the open hole. Having been involved in the very early development of the MeBo memory sondes, we used this successful example to develop, in cooperation with the same sonde manufacturer (ANTARES GmbH, Germany), a new and improved memory-logging sonde system motivated by a high demand for LWT capabilities in scientific drilling and a lack of affordable commercial LWT services.
The LWT system that we designed consists (from top to bottom) of a landing unit which can be adapted to different drill string dimensions, five combinable single memory sondes, and a precise, robust, and field-application-friendly depth-measuring device at the surface (Figs. 2, 3, 4).
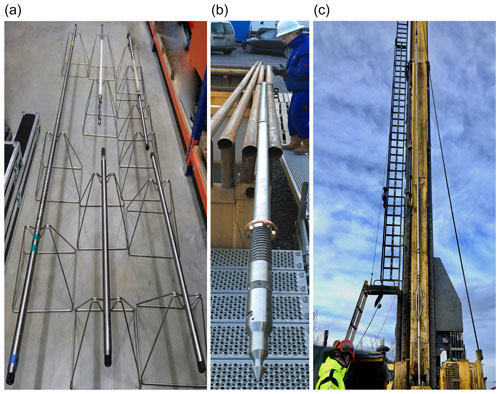
Figure 3Sondes of the ICDP memory-logging system (iMLS): (a) mBCS, mDIL, mMS, MemBat, mTS, and mSGR (clockwise from left). (b) Landing unit with fishing spear head, spiral spring shock absorber, and brass landing plate. (c) The completely assembled iMLS tool string hanging in a wireline drill derrick before being dropped into a HQ drill string down to the coring bit at 500 m.
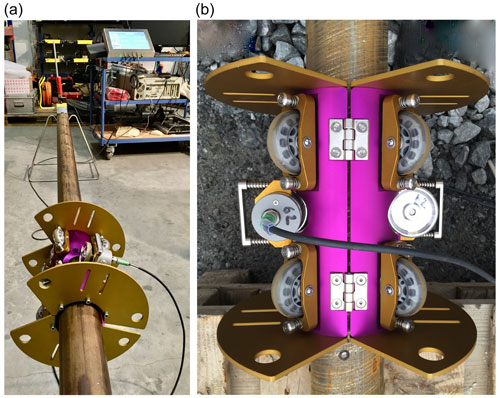
Figure 4(a) A photo of the testing of the depth-measuring system iDMD-W mounted on a HQ drill pipe under lab conditions. (b) A close-up of the iDMD-W. The two depth-counting wheels are in the middle on opposite sides, and four of the eight guide wheels are visible above and below, all mounted on the magenta-colored sleeve. The brass-colored side flanges are used to attach the iDMD to the rig.
3.1 The landing unit
This uppermost component of the iMLS tool string is mounted directly on the logging sondes. The purely mechanical landing unit is required to correctly position the actual measuring sondes inside and below the drill pipe. The unit consists of a fishing neck like that of an inner core barrel (Boart Longyear type), fitting pieces of different lengths, and a spring-loaded landing plate (Fig. 3). The modularity of the landing unit enables the use of the iMLS in various core drill strings with different bottom hole assembly geometry (outer core barrel and drill bit). Here, the length of the landing unit, the diameter of the landing plate, the hole size in the plate, and the stiffness of the impact suspension (spiral spring) are modified according to the length and the inner diameter of the outer core barrel. These exchanges can easily be done at the drill site, i.e., a change from PQ (bit size of 123 mm) to HQ (bit size of 96 mm) takes no more than 15 min. However, fitting rods for specific core barrel lengths have to be manufactured in advance. The iMLS is optimized for use in a wireline coring string with classic HQ dimensions (bit size of 96 mm), which is typical in ICDP drilling projects. With small adjustments, it can also be used in a larger PQ drill string (123 mm) and, with restrictions, in a smaller NQ string (76 mm). The suspension spring reduces the impact shock when the iMLS lands on the landing shoulder of the outer core barrel. Unlike an inner core barrel, which is anchored both downwards and upwards inside the outer core barrel, the landing plate does not latch; thus, the iMLS remains free upwards. This allows the sonde to give way upwards into the string should it accidentally be lowered and touch the well bottom or an obstruction. The descent rate of the sonde string inside the drill string is reduced by using an adapted landing plate design to change the flow resistance for a gentle and safe landing. The situation is different in strongly inclined bores with a deviation from vertical of > 50∘ where the weight of the sondes is usually no longer sufficient to overcome friction and to cause the sondes to stop sliding down the drill string. In such cases, the iMLS can be actively pumped down the pipe with the drill mud circulation until it lands on the drill bit.
3.2 The sondes
Based on proven wireline slimhole sondes, the iMLS sondes were further developed for autonomous memory measurements. These are so-called slimhole tools with a maximum outer diameter of 52 mm. All of the sondes can register at environmental conditions of up to 70 ∘C temperature and a maximum pressure of 50 MPa. This corresponds to a maximum depth of approximately 2–3 km in onshore drilling situations with a normal geothermal gradient.
Table 1Technical specifications. mSGR refers to the spectrum of the natural gamma radiation – total GR, K, U, and Th; mBCS refers to borehole-compensated sonic velocity – full waveforms, Vp and Vs; mMS refers to the magnetic susceptibility and temperature; and mDIL refers to electric resistivity and induction – Rmedium and Rdeep.
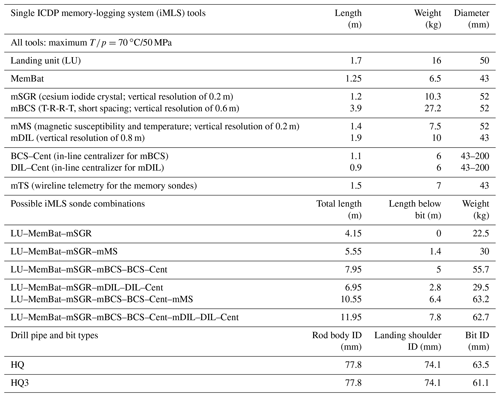
The iMLS instrument package includes the key geophysical and technical parameters desired most frequently in scientific drilling:
-
(MemBat) deviation from vertical – DEVI;
-
(mSGR) spectrum of the natural gamma radiation – total GR, K, U, and Th;
-
(mBCS) sonic velocity – full waveforms, Vp (Vs);
-
(mMS) magnetic susceptibility – MSUS and TEMP;
-
(mDIL) electric resistivity (induction) – Rmedium and Rdeep.
The minimum iMLS tool string is made up of the tool containing memory and battery (MemBat) and the mSGR tool, which is regularly crucial for precise core-to-log depth correction. The MemBat has a memory of 256 MB, equivalent to at least 500 h and provides a power supply of 12–15 VDC/24 Ah, equivalent to at least 50 h of recording with the maximum sonde stack. This is more than sufficient for every logging task within the depth range given by the tool specifications. The SGR sonde always remains positioned above the drill bit, i.e., within the drill pipe, because gamma rays do penetrate the steel pipe and the readings can be corrected for the attenuation. Sondes are typically combined in sonde stacks to minimize the number of logging runs and, hence, the required rig time. The minimum sonde stack of MemBat–mSGR can be combined with the other three sondes allowing for three longer sonde stacks: MemBat–mSGR–mBCS–mMS, MemBat–mSGR–mBCS–mDIL, and MemBat–mSGR–mMS (see Table 1).
The mSGR–mMS combination only protrudes 1.4 m out of the drill string bit and can, therefore, be used robustly in difficult borehole situations, even if the borehole is collapsed almost directly below the bit. The more fragile mDIL sonde is then only used in a second logging run, with the first run being utilized for hole condition reconnaissance. mBCS and mDIL are both used with in-line centralizers in the open hole. Another example of prioritization is lacustrine sediment logging with desired downhole parameters in a usual setup of (1) magnetic susceptibility and spectral gamma ray, (2) sonic velocity, and (3) electrical resistivity. Hence, the lowest risk choice for very difficult boreholes is the robust stack of mSGR and mMS; in less risky holes, the first run is the long stack mSGR–mBCS–mMS; and finally an mSGR–mDIL run may be utilized.
Besides being deployed using the drill string, the iMLS sondes can also be run in a borehole on any rope, slick line, or cable with a simple mechanical hook to still log in memory mode. It is a standard procedure to first run the mSGR alone on the coring wire line inside the drill string. This quickly yields a continuous total GR log that can be used for depth correlation of the logs from the subsequent LWT runs. The depth of this first log is determined independent of the driller depth-referenced LWT logs.
Furthermore, with a special additional wireline telemetry, the sondes can also be operated like normal logging sondes in wireline mode (online with real-time surface read-out). We made use of this additional feature in the field test and depth calibration test described below.
3.3 The iMLS depth-measuring device (iDMD)
In contrast to wireline logging, where a measuring wheel in the logging winch measures the length of the spooled cable directly while running the sondes in and out, the movement of the drill string first has to be measured precisely once it is tripped out for LWT. Several methods exist to do this. We developed two depth counters for the iMLS (1) because a ready-to-use depth device with LWT was not available on the market and (2) because we wanted to identify which of these two depth measurement methods is more practical, flexible, and robust under actual field conditions.
One depth counter works with a draw-wire sensor (iDMD-R, where R stands for rope) and the other works with measuring wheels (iDMD-W, where W stands for wheel). Both methods achieve an accuracy for the relative depth (length or traveled distance of the pipe movement) that compares very well to that of wireline logging (see below). However, the determination of the absolute LWT depth relies on the specification of the starting depth, i.e., the driller's depth of the bit before the start of the LWT. This depth is determined by the driller by measuring all drill string pieces on the pipe rack and adding them in the correct order during installation. Flawed pipe tallies cannot be excluded, meaning that the length of one or more pipe pieces may not have been counted, yielding a deeper bit position than the pipe tally indicates. As with any depth that is based on the driller's depth (e.g., bit depth, core depth, cuttings depth, and mud depth), the starting depth of the LWT also does not consider the lengthening of the drill string in the borehole due to its own weight. Accordingly, the obtained depth of the LWT is always less than the true depth. In the past, we have obtained differences between the wire line and driller's depth that varied by between almost zero in slim holes of only a few hundred meters depth and many meters in wide boreholes drilled for several kilometers with heavy pipe. However, during our new LWT measurements, the depth device reliably measured the true traveled distance of the already elongated drill string in the borehole.
3.3.1 iDMD-R (rope)
With this device, the movement of the drill string is measured indirectly via the movement of the hook or the top drive. For this purpose, a robust draw-wire encoder (Kübler D120) is attached in the drill rig so that the up and down movements of the hook or the top drive can be measured. As both could also be moved without actually moving the drill string, e.g., when repositioning the top drive, this must be considered in the depth measurement. Therefore, another sensor registers whether the lower rod holder is open (pipe hangs in the top drive, iMLS moves along, and measurement is registered) or is closed (pipe hangs in the rod holder, iMLS does not move, and no movement is registered). A similar depth systems is used for LWD and for the heave compensation in some marine drilling situations. The installation of a draw-wire depth device in a drill rig has to be carefully planned and tested so as not to interfere with the driller's activities. In our logging tasks, we do not always encounter the same rig and must instead contend with various drill rigs of very different sizes, constructions, and pipe handling types. Therefore, for the time being, we decided not to pursue this fragile and more difficult-to-adapt depth device, instead focusing on the development of a very small wheel-based device. However, we decided to keep our unperfected draw-wire device as a backup.
3.3.2 iDMD-W (wheel)
We designed the iDMD-W from scratch as a wheel-based depth measurement device that is basically comprised of two opposite wheels that are pressed directly onto the drill string (Fig. 4). In this sense, it measures very much like a wireline logging depth system that rides on the cable and records each movement of the cable at any time. These independent wheels continuously measure each movement and stoppage of the drill string passing through the depth device and, therefore, also those of the iMLS. Significant differences between the two readings make it possible to immediately recognize errors in the depth measurement. Errors could occur if a friction-reducing fluid caused the measuring wheels to occasionally slide on the drill string, which, in turn, would mean that the determined distances would be too short. In tests, one of the wheels was kept dry and the other wheel was moistened with water and with oil; these treatments did not cause any visible differences between the two length measurements. During measurement, the readings of both wheels are recorded and the larger value is chosen as the final depth output. This very compact and robust device is mounted like a sleeve around the drill string. The aluminum sleeve carries eight guide rollers and the two opposite measuring wheels. Due to its compactness, it can be installed in a position protected against damage between the top of the standpipe and the drill rod holder in a typical mining drill rig such as the ICDP DLDS (Deep Lakes Drilling System).
The technical functionality with respect to memory and wireline operation, pressure and temperature resistance, and handling of all iMLS sondes was verified in the 4000 m deep test borehole of the KTB Depth Laboratory (Harms and Kück, 2020).
The goal of the first full field utilization of the entire iMLS system was twofold: on the one hand, we aimed to check and improve the procedure that we had designed; on the other hand, we wished to examine the accuracy of the iMLS depth measurement method. The key operational objective was to exercise the complete LWT procedure for the first time, involving all components, and to compare the log depths obtained with those from subsequent wireline logging runs of the same memory sondes.
The field performance test was conducted during the active drilling operations at the 1000 m deep I-EDDA Test Center well (Almqvist et al., 2018). In cooperation with the drill rig operator, iMLS tests were carried out under realistic conditions in the depth range from 0 to 500 m. At the beginning of the test, the HQ core drill string was already installed in the hole with the coring bit at 500 m. First, a mechanical test was carried out with a dummy sonde, consisting of the landing unit and a solid steel bar with the dimensions and weight of an iMLS sonde string. After confirming the resilience and retrievability of the landing unit, the actual iMLS logging in memory mode was carried out along the depth section from 500 to 390 m with the long sonde combination of LU–MemBat–mSGR–mBCS–BCS–Cent–mMS (Fig. 3). A wheel-based depth counter prototype and the draw-wire device were used one after the other. The iDMD-W described above was not yet available at that time. The large size of the wheel-prototype only allowed for a mounting position between the rod holder and the top drive. Unfortunately, the prototype was rammed by the downward-moving top drive after only 8 m of pipe tripping and became unusable, whereupon the iDMD-R was used. After the complete trip-out of the drill string, we ran the iMLS sondes in wireline mode in the open hole using a 600 m logging winch. We ran the same sonde stack as that used in LWT memory mode for the wireline mode logging. As the sondes and the logging speed were identical in memory and wireline logging, the logs show the same values and amplitudes, although their depth positions may have differed due to the very unequal depth-measuring method. The direct comparison of memory and wireline logs was then used to estimate the accuracy of the memory depth. The depth of the wireline log can be assumed to be almost equal to true depth because the TC1 hole is vertical and has a very smooth wall surface at these shallow depths.
When comparing wireline and memory logs, the memory depth first had to be shifted by a constant value using simple visual correlation due to the inexact starting depth information (drill bit depth) of the memory registrations. The magnetic susceptibility log (MSUS) was best suited to this task due to the almost complete reproduction of its profile in repeat measurements and its high vertical resolution of less than 0.1 m. Depth offsets for correction to the wireline depth were calculated as +4.6 m for the wheel prototype and +8.8 m for the iDMD-R. After this correction of the starting depth, the comparison of the wireline and the memory logs shows a remarkably good agreement of the depth of the measured curves (Fig. 5). The logs are only used here for the purpose of depth correlation. A detailed evaluation of these and additional geophysical logs along with core-derived data is the subject of ongoing investigations.
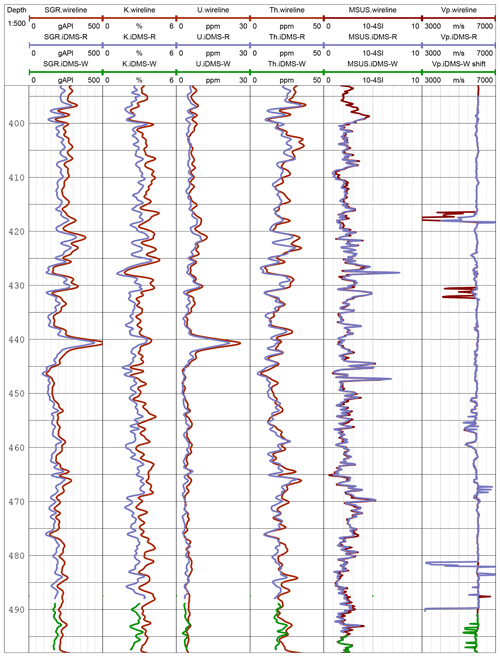
Figure 5Depth (in meters) comparison of iMLS logging data from the wireline mode run (brown) and the memory mode run with two independent depth devices (green represents the iDMD-W prototype, and blue represents the iDMD-R) in the TC1 well, Örebro, Sweden. Depth differences between the wireline and memory mode are less than 0.1 m. Here, SGR denotes the parameter total gamma ray measured by the spectrum GR tool, and K, U, and Th are the contents of potassium, uranium, and thorium, respectively. All mSGR-derived curves are corrected for the effects of borehole size and steel casing. MSUS is the magnetic susceptibility, and Vp is the sonic P-wave velocity.
The excellent depth match is most evident in the high-depth-resolution curves of the magnetic susceptibility (MSUS), which almost overlay each other. The average depth difference is 0.2 m, which is in the order of the sample spacing of 0.1 m. Furthermore, the four parameters of the natural gamma ray spectrum sonde mSGR (total SGR; potassium, K; uranium, U; and thorium, Th) show a very good depth correlation of memory and wireline curves. All mSGR curves are corrected for the small hole size, and the memory curves are additionally corrected for the attenuation by the drill pipe. Slight differences in the curve shapes are due to the statistical nature of the natural gamma radiation as a product of radioactive decay. The P-wave velocity curves (Vp) of the sonic tool also show a good log–log correlation except for small divergences in a few short zones resulting from the difficult-to-process sonic waveform data.
The depth differences are less than 0.1 m along the 100 m long section. This demonstrates the ability of the iMLS depth devices to determine the movement of the sonde string in the borehole with the same accuracy and resolution as the classic wireline method. However, it also shows how immensely important the reliability of the exact starting depth reported by the driller is for LWT, without which an absolute LWT depth determination is impossible; for example, in lake drilling projects, the drop in the total GR at the lake floor can sometimes be used as a reference, whereas in onshore drilling situations, this is not the case. As described above, differences of up to several meters between the bit depth notified by drillers and the wireline logger depth are common. For this reason, whenever possible, a wireline log of total gamma ray and magnetic susceptibility should be carried out before an iMLS run. Even if only a short MSUS log length in the open hole is possible, the gained short profile can be used for depth correlation. A single total gamma ray would allow for a correlation, albeit a coarser one, in a case where a wireline sonde cannot run out of the bit at all. Logistically, a wireline pre-measurement will be possible in cases where the iMLS serves as a backup for the primary preferred wireline measurements. This procedure is not possible in strongly inclined bores. Ultimately, even without an absolute depth reference (e.g., wireline depth), the LWT depth will instantaneously match the core depth, as both refer to the driller's depth.
Although our system is now basically ready for regular use in HQ and PQ holes, we will use both our iMLS and wireline logging systems in upcoming projects to carry out further tests of the depth-recording device in comparison to wireline logging in order to improve the iMLS performance and to verify the observed accuracy of the memory depth measurement on a broader statistical basis.
This report is a technical description of the ICDP memory-logging system. For information on the availability of the iMLS and conditions for use see the SUPPORT section of the ICDP website (https://www.icdp-online.org/support/service/downhole-logging/operational-support/, last access: 17 April 2021). Logging data presented in this report are preliminary and are not yet publicly available, as they are still being evaluated. Upon completion, all data will be made available when the associated scientific papers and reports are published.
JK planned and led this project and all field tests. MG designed the landing unit, the depth devices, and the software. AJ provided machine drawings of the landing unit and the wheel depth device. MG and MT tested and improved the entire system in the lab and in the field tests. JK and UH managed the project and designed the paper. All authors contributed to the paper.
The last author is editor-in-chief of Scientific Drilling; all other authors declare that they have no conflict of interest.
Any use of trade, firm, or product names is for descriptive purposes only.
We thank Jan-Erik Rosberg, Johan Kullenberg, Simon Rejkjär, and Peter Jonsson from the University of Lund, Sweden, for their patience and tireless help with preparing and undertaking the field test. The reviews of Gilles Guerin and David Goldberg contributed to the improvement of this report.
Development, manufacturing, and acquisition of the iMLS were funded by the International Continental Scientific Drilling Program, ICDP. The field campaign has been funded by the EIT Raw Materials project: Innovative Exploration Drilling and Data Acquisition Test Center – I-EDDA-TC (grant agreement EIT/RAW MATERIALS/SGA2019/1).
This paper was edited by Tomoaki Morishita and reviewed by David Goldberg and Gilles Guerin.
Abbott, M. B. and Rodbell, D. T.: Stratigraphic correlation and splice generation for sediments recovered from a large-lake drilling project: an example from Lake Junín, Peru, J. Paleolimnol., 63, 83–100, https://doi.org/10.1007/s10933-019-00098-w, 2020.
Aivalis, J., Meszaros, T., Porter, R., Reischman, R., Ridley, R.,Wells, P., Crouch, B. W., Reid, T. L., and Simpson, G. A.: Logging Through the Bit, Oilfield Review Summer 2012, 24, 44–53, 2012
Almqvist, B., Brander, L., Giese, R. Harms, U., Juhlin, C., Lindén, C., Lorenz, H., and Rosberg, J.: I-EDDA test center for core-drilling and downhole investigations, EGU General Assembly 2018, 8–13 April 2018, Vienna, Austria, Geophysical Research Abstracts, 20, EGU2018-14837, 2018.
Baumgarten, H. and Wonik, T.: Cyclostratigraphic studies of sediments from Lake Van (Turkey) based on their uranium contents obtained from downhole logging and paleoclimatic implications, Int. J. Earth Sci., 104, 1639–1654, https://doi.org/10.1007/s00531-014-1082-x, 2015.
Beal, J.: Tight oil vertical log analysis applied to horizontal Logging While Tripping (LWT) data of Cretaceous-aged Viking formation, Saskatchewan, Canada: a multi-disciplinary review of initial and extended findings, AAPG Rocky Mountain Section Meeting, Cheyenne, WY, USA, 2019.
Freudenthal, T., Bohrmann, G., Gohl, K., Klages, J. P., Riedel, M., Wallmann, K., and Wefer, G.: More than ten years of successful operation of the MARUM-MeBo sea bed drilling technology: Highlights of recent scientific drilling campaigns, EGU General Assembly, Online Conference, 4–8 May 2020, SSP1.4, 2020.
Gebhardt, A. C., Francke, A., Kück, J., Sauerbrey, M., Niessen, F., Wennrich, V., and Melles, M.: Petrophysical characterization of the lacustrine sediment succession drilled in Lake El'gygytgyn, Far East Russian Arctic, Clim. Past, 9, 1933–1947, https://doi.org/10.5194/cp-9-1933-2013, 2013.
Goldberg, D.: The Role of Downhole Measurements in Marine Geology and Geophysics, Rev. Geophys., 35, 315–342, https://doi.org/10.1029/97RG00221, 1997.
Guerin, G. and Goldberg, D.: Heave compensation and formation strength evaluation from downhole acceleration measurements while coring, Geo-Mar. Lett., 22, 133–141, https://doi.org/10.1007/s00367-002-0104-z, 2002.
Hansen, R. R. and White, J.: Features of Logging-While-Drilling (LWD) in Horizontal Wells, SPE/IADC Drilling Conference, 11–14 March 1991, Amsterdam, the Netherlands, 21989-MS, https://doi.org/10.2118/21989-MS, 1991.
Harms, U. and Kück, J.: KTB Depth Laboratory: A Window into the Upper Crust, in: Encyclopedia of Solid Earth Geophysics, Encyclopedia of Earth Sciences Series, edited by: Gupta, H. K., https://doi.org/10.1007/978-3-030-10475-7_242-1, 2020.
Hodell, D., Anselmetti, F., Brenner, M., Ariztegui, D., and the PISDP Scientific Party: The Lake Petén Itzá Scientific Drilling Project, Sci. Dril., 3, 25–29, https://doi.org/10.2204/iodp.sd.3.02.2006, 2006.
Jackson, M. D., Gudmundsson, M. T., Weisenberger, T. B., Rhodes, J. M., Stefánsson, A., Kleine, B. I., Lippert, P. C., Marquardt, J. M., Reynolds, H. I., Kück, J., Marteinsson, V. T., Vannier, P., Bach, W., Barich, A., Bergsten, P., Bryce, J. G., Cappelletti, P., Couper, S., Fahnestock, M. F., Gorny, C. F., Grimaldi, C., Groh, M., Gudmundsson, Á., Gunnlaugsson, Á. T., Hamlin, C., Högnadóttir, T., Jónasson, K., Jónsson, S. S., Jørgensen, S. L., Klonowski, A. M., Marshall, B., Massey, E., McPhie, J., Moore, J. G., Ólafsson, E. S., Onstad, S. L., Perez, V., Prause, S., Snorrason, S. P., Türke, A., White, J. D. L., and Zimanowski, B.: SUSTAIN drilling at Surtsey volcano, Iceland, tracks hydrothermal and microbiological interactions in basalt 50 years after eruption, Sci. Dril., 25, 35–46, https://doi.org/10.5194/sd-25-35-2019, 2019.
Jerram, D. A., Millett, J. M., Kück, J., Thomas, D., Planke, S., Haskins, E., Lautze, N., and Pierdominici, S.: Understanding volcanic facies in the subsurface: a combined core, wireline logging and image log data set from the PTA2 and KMA1 boreholes, Big Island, Hawai`i, Sci. Dril., 25, 15–33, https://doi.org/10.5194/sd-25-15-2019, 2019.
Koeberl, C., Milkereit, B., Overpeck, J. T., Scholz, C., Amoako, P. Y. O., Boamah, D., Danuor, S., Karp, T., Kück, J., Hecky, R. E., King, J. W., and Peck, J. A.: An international and multidisciplinary drilling project into a young complex impact structure: The 2004 ICDP Bosumtwi Crater Drilling Project – An overview, Meteorit. Planet. Sci., 42, 483–511, 2007.
Matheson, R. and West, J.: Logging While Tripping – A New Alternative in Formation Evaluation, J. Can. Petrol. Technol., 39, 38–43, https://doi.org/10.2118/00-07-02, 2000.
Moore, J. C.: Synthesis of results: logging while drilling, northern Barbados accretionary prism, in: Proc. ODP, Sci. Results, 171A: College Station, TX (Ocean Drilling Program), edited by: Moore, J. C. and Klaus, A., 1–25, https://doi.org/10.2973/odp.proc.sr.171a.101.2000, 2000.
Singh, M., Al Benali, K., A., Sallam, Y., Sajeel, K., El Wazeer, F., Chaker, H., and Propper, M.: A Case Study on Open-Hole Logging While Tripping LWT Through Drill Pipes, as a New Technology for Risk Mitigation and Cost Optimization in Abu Dhabi Onshore Fields, Society of Petroleum Engineers, SPE-193315-MS, https://doi.org/10.2118/193315-MS, 2018.