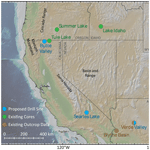
Workshop report: PlioWest – drilling Pliocene lakes in western North America
Alison J. Smith
Emi Ito
Natalie Burls
Leon Clarke
Timme Donders
Robert Hatfield
Stephen Kuehn
Andreas Koutsodendris
Tim Lowenstein
David McGee
Peter Molnar
Alexander Prokopenko
Katie Snell
Blas Valero Garcés
Josef Werne
Christian Zeeden
The Pliocene Epoch is a focus of scientific interest as a period of sustained global warmth, with reconstructed CO2 concentrations and a continent configuration similar to modern. Numerous studies suggest that the Pliocene was warmer and largely wetter than today, at least in the subtropics, which contrasts with the long-term hydroclimatic response of drying conditions predicted by most climate model simulations. Two key features of Pliocene warmth established from sea surface temperature reconstructions could affect dynamic changes that influence the hydrologic cycle: (1) a weaker Pliocene zonal gradient in sea surface temperature (SST) between the western and eastern equatorial Pacific resembling El Niño-like conditions and (2) polar-amplified Pliocene warmth, supporting a weaker Equator-to-pole temperature gradient. The distribution of wet conditions in western North America and the timing of late Pliocene–Quaternary aridification offer the potential to evaluate the relative roles of these two external forcings of the climate in western North America, with broader global implications for Mediterranean-type climate (MTC) regions. We convened a virtual ICDP workshop that spanned a 2-week period in September 2021, to choose optimal drill sites and legacy cores to address the overall scientific goals, flesh out research questions, and discuss how best to answer them. A total of 56 participants from 12 countries (17 time zones), representing a wide range of disciplines, came together virtually for a series of plenary and working group sessions. We have chosen to study five basins (Butte Valley, Tule Lake, Lake Idaho, Searles Lake, and Verde Valley) that span 7 ∘ of latitude to test our hypotheses and to reconstruct the evolution of western North American hydroclimate with special focus on the time ranges of 4.5–3.5 and 3–2.5 Myr. Although individual Pliocene lake records occur in many areas of the world, the western North American basins are unique and globally significant as deep perennial freshwater Pliocene lakes latitudinally arrayed in a MTC region and are able to capture a response to Pacific forcing. We propose new drill cores from three of these basins. During the workshop, we discussed the stratigraphy and subsurface structure of each basin and revised the chronological frameworks and the basin-to-basin correlations. We also identified the best-suited proxies for hydroclimate reconstructions for each particular basin and put forward a multi-technique strategy for depth–age modeling. Reconstructions based on data from these sites will complement the SST reconstructions from global sites spanning the last 4.5 Ma and elucidate the large-scale hydrological cycle controls associated with both global warming and cooling.
- Article
(6641 KB) - Full-text XML
- BibTeX
- EndNote
The Pliocene Epoch has become the focus of scientific interest as a period of sustained global warmth. With reconstructed CO2 concentrations in the range of 350–450 ppm and a continent configuration similar to modern, the Pliocene is an appropriate analogue for testing the capabilities of numerical models used to predict climate and ecological change over the coming century. An array of geologic studies now suggests that the Pliocene was warmer and largely wetter, at least in the subtropics, than today, which contrasts with the long-term hydroclimatic response seen in most climate models in response to elevated CO2 (Molnar and Cane, 2007; Ibarra et al., 2018; Lepar and Firk, 2015; Lukens et al., 2019). But what caused wetter conditions in subtropical Mediterranean-type climate (MTC) regions (Rundel et al., 2016) in the Pliocene, when theory and model simulations indicate that these regions become drier with global warming? Where were those sources of precipitation in these MTC regions (Rundel et al., 2016; Fu et al., 2022), and how did the hydroclimatic regime change with the onset of Pleistocene glacial–interglacial cycles? Answering these and related questions requires a spatial gradient of high-resolution multiproxy terrestrial paleoclimate records that are central to ICDP's Research Plan for 2020–2030 in the focus area of environmental change and water resources.
Understanding the Pliocene hydrological cycle is a target of the PlioWest project, as our ability to predict future hydroclimatic conditions may be limited by how well we replicate these conditions during Pliocene peak warmth. We convened a virtual ICDP workshop that spanned a 2-week period in September 2021, to choose optimal drill sites and legacy cores to address the overall scientific goals, flesh out research questions, and discuss how best to answer them. The overarching plan of this workshop, to bring together an international group of scientists to work collaboratively on how best to approach the question of causal mechanisms of wetter conditions in the Pliocene in MTC regions, was successfully achieved despite the less-than-optimal, virtual meeting format.
The 2020–2021 global pandemic blocked our abilities to offer an in-person international workshop in September 2020. After a year's postponement, we had hoped to offer a hybrid workshop, with online sessions and an in-person component to take place in Minneapolis, Minnesota, at the CSD Core Lab facility. That model was also necessarily abandoned as the pandemic persisted, and we embarked on an entirely virtual workshop for 56 registrants from 12 countries, following advertisement of the workshop with AGU and ICDP (Fig. 1). The workshop took place as a series of meetings using Zoom and was completed over the month of September 2021 in 90 min sessions, co-chaired by Alison J. Smith (Kent State University) and Emi Ito (University of Minnesota). The workshop consisted of three plenary sessions, with the first two plenaries each separated by 10 discussion group sessions of 60–90 min length. These discussion groups were separately scheduled events, with no more than six discussants in any session. Plenary sessions included a few invited presentations followed by verbal summaries. All notes, presentations, site data, and other relevant material were accessible to the registrants through a web-based communication site. In the final plenary session, consensus was reached on the overall strategy and drill sites to be studied, and a development timeline and action items were summarized about the next steps towards completion of a compelling full ICDP proposal. Focus groups were then identified to continue specific work via Zoom meetings towards the completion of a drilling proposal for submission to the ICDP. Because a seismic survey for one of the basins needs to be done, we are working toward the January 2024 drilling proposal deadline.
Why the Pliocene was warmer and, in some regions, wetter than conditions predicted for the end of this century is a challenging question with many societal implications. The primary mechanism leading to future projected drying in Southern California, and other MTC regions, is a thermodynamically driven increase in the moisture transport divergence from these regions (Seager et al., 2010; Seager and Vecchi, 2010). This mechanism follows a simple thermodynamic argument for how the hydrological cycle might respond to increases in global temperature given the Clausius–Clapeyron relation, whereby warm air can hold more moisture than cold air (Held and Soden, 2006) (Fig. 2a). While limitations exist in this first-order argument over land (Byrne and O'Gorman, 2015), climate model projections (Seager et al., 2010; Seager and Vecchi, 2010), and near-equilibrium simulations in which CO2 is abruptly increased (Fig. 2a, c, e), do indeed simulate the general drying of Mediterranean climate zones.
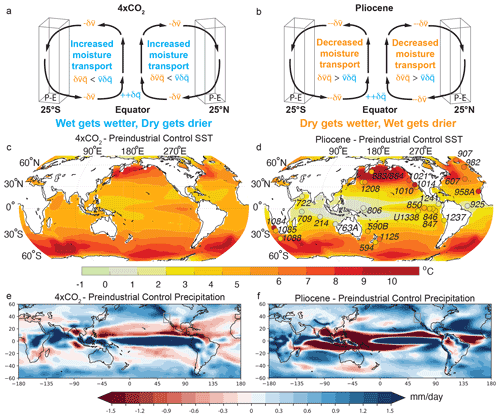
Figure 2An illustration of the competition between thermodynamically forced subtropical drying due to a global mean temperature increase and the dynamically forced subtropical wetting due to weakening surface temperature gradients and hence atmospheric circulation, most notably the Hadley atmospheric overturning circulation, schematically shown in (a) and (b). While there is some weakening of gradients, under 4 × CO2 (4 × 280 ppm) (c, e), the global mean temperature change dominates (relatively speaking), leading to either reduced or nearly unchanged annual mean precipitation in MTC regions. A strong reduction in gradients during the Pliocene leads to wetter conditions in most MTC regions (d, f) despite global warming. The superimposed circles in panel (d) indicate Early Pliocene sea surface temperature reconstructions, portrayed on a surface consistent with Early Pliocene proxy data. Adapted by Natalie Burls from Burls and Fedorov (2017, Figs. 1 and 4).
Substantial changes in atmospheric circulation (Burls and Federov, 2017; Fu et al., 2021; Menemenlis et al., 2022), which this simple thermodynamic expectation neglects, could however act to counter subtropical drying and lead to the opposite response – wetter subtropical conditions (Fig. 2b, d, f). A paired latitudinal and longitudinal transect of records as proposed here is the only research strategy able to test these atmospheric circulation hypotheses by capturing the north–south and east–west hydroclimatic gradients and the temporal and spatial extent of these changes in moisture delivery.
Pliocene surface temperature reconstructions show two key features that climate models struggle to reproduce. These have the potential to bring about atmospheric circulation changes that could have supported wetter conditions in western and other MTC regions across the globe.
One such feature is a Pliocene zonal gradient in sea surface temperature (SST) between the western and eastern equatorial Pacific that is weaker than the present day, resembling El Niño-like conditions (e.g., Chaisson and Ravelo, 2000; Wara et al., 2005; Groeneveld et al., 2006; Lawrence et al., 2006; Ravelo et al., 2006; Dekens et al., 2007; Herbert et al., 2016). The atmospheric response to modern El Niño events leads to an equatorward shift and eastward extension of the subtropical jet, a strengthened Aleutian Low, and heavier winter rainfall over the southwestern but not the northwestern USA (e.g., Schonher and Nicholson, 1989; Trenberth et al., 1998; Seager et al., 2010), with a somewhat similar response projected for the future in models that include a more El Niño-like mean state (Allen and Luptowitz, 2017). It follows that lakes, at least in part of the southwestern USA, might have resulted from atmospheric circulation patterns associated with a permanently warm eastern tropical Pacific during the Pliocene. Following the modern association, one might predict that a permanent El Niño-like Pliocene SST distribution supported an enhancement of today's winter rainfall patterns (Molnar and Cane, 2002, 2007; Winnick et al., 2013; Ibarra et al., 2018) but with more weighting towards central-southern California and less over the Pacific Northwest, following the dipole nature of the modern climate teleconnection.
A second feature is polar-amplified Pliocene warmth, supporting a weaker Equator-to-pole (meridional) temperature gradient (Dowsett et al., 2012), specifically between the tropics and the midlatitudes (e.g., Brierley et al., 2009; Fedorov et al., 2015), as this scales with Hadley strength. The reduced meridional temperature gradient during the Pliocene offers a mechanism for creating wetter subtropics globally (Burls and Fedorov, 2017); by supporting a weaker Hadley circulation, it allows for a reduction in the divergence of moisture from the arid subtropics that overcomes the thermodynamic enhancement that occurs with global warming, giving rise to dry-gets-wetter conditions in the subtropics (Fig. 2b, d). For the western USA, climate simulations with a strongly reduced Equator-to-pole (and equatorial zonal) gradient support wetter conditions in Southern California through more summer rainfall, as well as to the north through increased winter rainfall (Fig. 2f, Burls and Fedorov, 2017). Thus this mechanism might predict wetter conditions for both the northern and southern sites in this proposed study.
Reconstructing the distribution and seasonality of wet conditions in western North America and the timing of late Pliocene–Pleistocene aridification offers the potential to evaluate the role of these two mechanisms. Evidence suggests that they were likely linked (Fedorov et al., 2015) and therefore working in tandem. Our working hypothesis is that the hydroclimate of western North America was, in addition to global warmth, dictated by changes in the strength of Pacific zonal and meridional surface temperature gradients. We plan to drill cores from three of these basins (see Sect. 4, “Proposed drilling sites”) in order to reconstruct the evolution of the western North American hydroclimate, with special focus on the time ranges from 4.5–3.5 and 3–2.5 Ma. Focus on these time ranges will bring attention to the Early Pliocene hydroclimatic conditions and also to the later onset of the glacial–interglacial cycle and intensification of Northern Hemisphere glaciation.
We have created a synthetic time series of precipitation estimates for the northern Butte Valley region (Fig. 3a) and southern Searles Lake and Verde Valley region (Fig. 3b) to explore how our working hypotheses can be tested. This initial analysis suggests that the precipitation history should have varied to differing extents in the subtropical vs midlatitude portions of the study region and could be compared with the multiproxy records from the proposed array of lake coring sites.
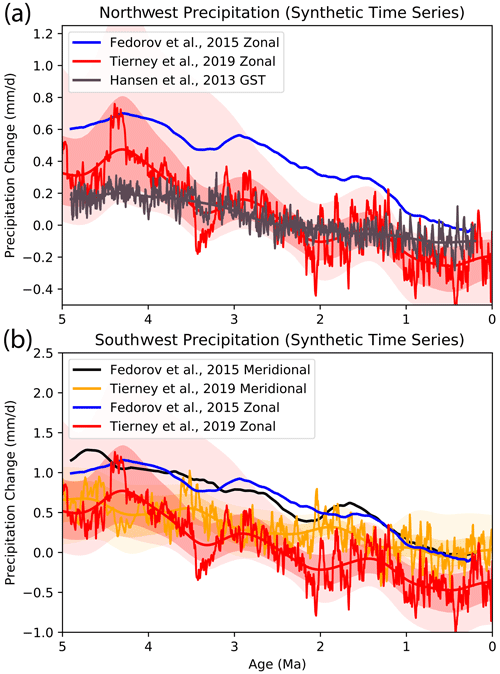
Figure 3Synthetic time series of (a) northwest (41–45∘ N, 122–115∘ W) and (b) southwest (31–35∘ N, 118–114∘ W) annual mean precipitation change relative to preindustrial (or 0–0.5 Ma) (in mm per day) derived as a function of the zonal and meridional SST gradient reconstructions presented in Fedorov et al. (2015) and Tierney et al. (2019) (the light shading represents the full range and the darker shading the 95 % uncertainty range provided for the gradient estimates of Tierney et al., 2019), as well as the global mean surface temperature reconstruction of Hansen et al. (2013). Synthetic time series were generated using the relationships (statistically significant at the 95 % confidence interval) between regional precipitation and the zonal and meridional SST gradients, respectively, as well as global mean temperature, across the 30, fully coupled, global climate model experiments (Burls and Fedorov, 2017). These results suggest the southern drill sites should exhibit more Pliocene “wetting” relative to pre-industrial than the northern sites (proposed drill site and legacy cores included), as well as be more sensitive to both zonal and meridional SST gradient changes (more dynamically driven), as opposed to the northern site, which should display more sensitivity to global mean temperature (more thermodynamically driven). Figure created by Natalie Burls.
Additional hypotheses to be tested include the following:
-
Pliocene warmth led to systematic changes in precipitation seasonality and extremes.
-
Establishment and later disappearance of large freshwater ecosystems profoundly affected the establishment of and subsequent dispersal, extirpation, or extinction of fauna and flora.
-
Marine Isotope Stages (MIS) M2 and KM2 events were of sufficient magnitude and duration to be recorded in these MTC lake sediments.
Although Pliocene lake basins occur in several subtropical and MTC regions worldwide (Mediterranean Basin, Southwestern Australia, Chile, California, China, and others), western North America contains the only set of deep, permanent lake basins that span several degrees of latitude and that existed during most of the Pliocene Epoch. Western North America contains accessible and under-recognized paleolacustrine records that hold the keys to understanding the drivers of wetter conditions in Pliocene subtropical drylands worldwide.
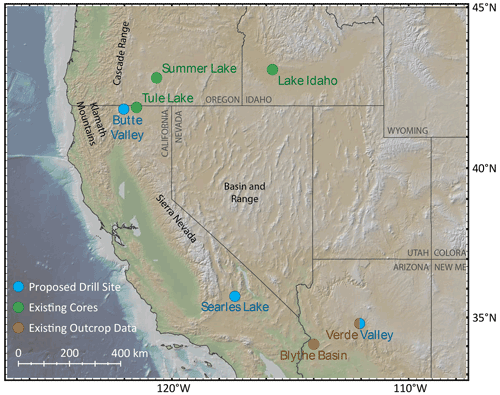
Figure 4Location map of proposed drill sites and sites with existing sediment core and outcrop material available for study from previous drilling campaigns. Existing material from Blythe Basin and Verde Valley is from outcrop studies only. The Summer Lake records are short cores. Searles Lake and Butte Valley each have a Plio-Pleistocene core (see Fig. 5). All sites were discussed and evaluated during the workshop. The Cascade Range, Klamath Mountains, Sierra Nevada, and the Basin and Range are also shown. Figure created by Robert Hatfield, made with GeoMapApp (http://www.geomapapp.org, last access: 29 December 2021)/CC BY (Ryan et al., 2009).
Going into the workshop, we had an array of possible drilling sites, identified during a smaller previous EarthRates-funded workshop. These included Summer Lake, Oregon; Lake Idaho, Idaho; Butte Valley, California; Tule Lake, California; Searles Lake, California; and Blythe Basin, Arizona (Fig. 4). Discussion focused on the previous work at these sites, the likelihood of recovering continuous sediments reaching to at least 4.5 Ma, the presence/absence of carbonates and organic biomarkers, site access, available subsurface data for robust site characterizations, and the likelihood of developing depth–age models and robust correlation models across the study region. We learned that Blythe Basin was likely to capture only the Lower Pliocene, which led to a discussion of possible replacement sites, leading us to discuss Verde Valley, Arizona, a site in central Arizona that had no drilling history but a record of outcrop studies. We reached consensus towards the end of the workshop to drill four sites which showed great promise: Lake Idaho, Butte Valley, Searles Lake, and Verde Valley. This plan was later altered to three drill sites, based on new dating information from Lake Idaho, which indicated the paleolacustrine record at Mountain Home drill site was no older than about 3.1 Ma (Alexander Prokopenko, personal communication, December 2021). The older sediments of Lake Idaho are likely to be found farther west and lie beneath thick layers of basalt making site survey uninformative and costly. The final list of drill sites for the project included Butte Valley and Searles Lake, California, and Verde Valley, Arizona (Figs. 4 and 5). We also intend to study the existing USGS legacy cores from Butte Valley, Tule Lake, Lake Idaho (USGS–Bruneau Core), and Searles Lake and to collaborate with the ICDP HOTSPOT team that recovered the Plio-Pleistocene paleolacustrine record from Mountain Home, Idaho (Figs. 4 and 5). Combined, our sites comprise a north–south transect covering about 7∘ in latitude (1380 km), to capture the changing hydroclimatic gradients over time. Both northern and southern sites consist of lake records with ∼ 600 km E–W distance between them, enabling us to examine the extent and duration of westerly moisture transport across this north–south transect.
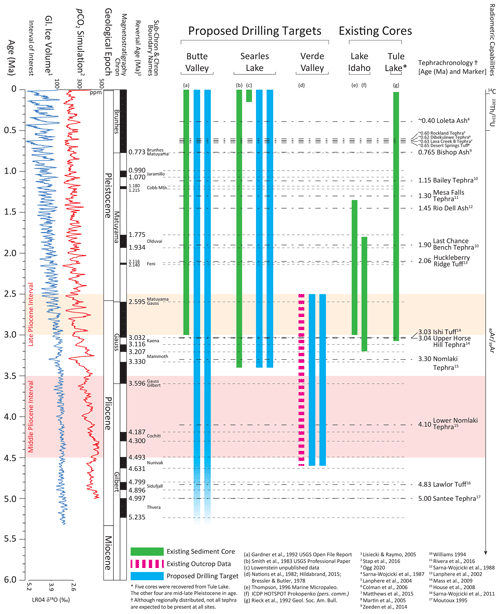
Figure 5Preliminary model for correlating and dating the proposed sites. Time ranges dateable by 14C, 230Th 234U, and 40Ar 39Ar radiometric methods are indicated at the right-hand edge. Magnetostratigraphy (chrons and subchrons) is shown to the left. Figure created by Robert Hatfield with input from Stephen Kuehn.
Our science team will use an integrated strategy in analyzing the complex story of paleoclimate and paleoecology in these Pliocene and Plio-Pleistocene lake sediment records of western North America. The strategies are described in brief here. The range of scientific expertise represented by our project team is shown in Fig. 1b.
5.1 Chronology
We will combine multiple dating techniques to maximize temporal precision and coverage, as well as robust correlations between paleolakes. The underlying framework for this chronology will be improved accuracy of depth and stratigraphic information. Core depths will be tied to logging depth through common gamma ray data obtained during downhole logging and from core-scanning measurements. Where available, overlapping cores from multiple holes at the same site will be correlated to generate a composite depth scale and continuous stratigraphic section (e.g. Hatfield et al., 2020). Using this foundation, depth will be mapped to time using a suite of methods, primarily through paleomagnetic polarity stratigraphy and tephrostratigraphy supported by biostratigraphy (pollen and spores), radiometric dating (uranium–thorium, argon–argon, beryllium-10), and, where available, orbital and/or climatic tuning. The multiproxy integrated age–depth model will be generated using Bayesian approaches (e.g. Lougheed and Obrochta, 2019) that allow for realistic estimates of uncertainty.
5.2 Climate and environmental proxies
Paleobiological analyses, augmented by organic and inorganic geochemical proxies, are key components of the planned overarching post-coring scientific measurements. We aim to reconstruct both the (i) paleoclimatic evolution and (ii) biotic response to climatic and geological forcing. Biological proxies include pollen, diatoms and ostracodes, and geochemical/biochemical proxies include leaf-wax hydrogen isotopes, algal lipid δ2H, and δ13C and δ18O from inorganic and biogenic carbonates. Wildfire, an important part of terrestrial ecosystems in the study region, will be studied through analysis of such proxies as charcoal, PAHs (polycyclic aromatic hydrocarbons), δ13C in leaf waxes, and phytoliths. Analyses will be carried out at different temporal resolutions in order to identify both tectonic to orbital timescale trends and suborbital to sub-millennial timescale variability to produce datasets that are most relevant to present-day anthropogenic climate and ecosystem change.
PlioWest has clear links to water security and landscape change, which are ongoing topics of increasing interest to all stakeholders in western North America, including Native American tribal communities, watershed managers, and agricultural planners. Development of educational outreach opportunities involving these topics will be an important aspect of the NSF and other funding agency proposals. Although the proposed drill sites are all situated on federal land (with the exception of Searles Lake, on privately owned land), these lands and the environments they represent are part of Native American cultural heritage. We will involve key stakeholders (local schools, colleges, public libraries, state and national parks, museums, businesses, and tribal representatives) at the early stages of project planning to learn what information we can gather that is important for them and their communities. This method, known as community-driven research (CDR; Pandya, 2014, and the mission of AGU's Thriving Earth Exchange), has been shown to increase community buy-in and trust in the results of the research. We will further develop these plans in consultation with the CSD Facility and with the Geoscience Alliance, an NSF-funded organization whose goal is to broaden participation of Native Americans in geosciences. This approach dovetails with a growing area of co-production of research (Zurba et al., 2022), and we look forward to opportunities to work collaboratively in this way with tribal communities.
Additionally, by working with the public access community database Neotoma (https://www.neotomadb.org/, last access: 28 November 2022), data and educational tools will become available to the public for use in educational settings at many levels, from secondary education through university level. Finally, we plan to create openly available educational videos throughout the project, following in the path of impactful examples such as the ANDRILL project (https://www.wgtn.ac.nz/antarctic/research/past-research-prog/andrill, last access: 28 November 2022), which can serve secondary school and university students, as well as the public.
By recovering continuous core records from the targeted paleolake sites, we expect to gain insights into those step changes that occurred during the Early Pliocene warm period and subsequent Pleistocene cooling that accompanied the hydroclimatic changes in this MTC region. The mechanisms involved may have led to systematic changes in rainfall seasonality and extremes and may have changed the ecology of the entire region. Gaining new knowledge about these interlinked changes will certainly impact those working in the areas of climate change, water and food security, and ecological and environmental sciences. Climate extremes, beyond those of present-day climate, offer opportunities to test climate models used to predict future climate scenarios. The possible mismatch of predictions of a drier future climate for MTC regions, including the western USA, epitomizes how understanding the wet Pliocene can show how paleoclimate can be used to test and improve models and thus understanding of the global system that has direct societal relevance. The outcome of this study will benefit climate modelers, policy makers, and the many communities that make use of those models.
We also note that the virtual ICDP workshop format is doable and can achieve completion of a full ICDP proposal but is not the most desirable means of conducting a scientific workshop. The weak point in this format is the difficulty of generating an environment that produces spontaneous scientific discussions and a spirit of camaraderie, as well as the logistical challenges of drawing multiple individuals together when subject to their individual commitments. We were able to achieve our goals over a more expanded time frame (multiple meetings over a 2-week period) and with meticulous planning and constant adjustments to those plans by the two convenors of the workshop (Alison J. Smith and Emi Ito). Of course, the key benefit of a virtual workshop was much reduced cost as we had no travel and accommodation costs, but in the end, we found there is no substitute for in-person scientific workshops, and we look forward to the imminent return of such events.
No datasets were used in this article.
PlioWest Working Consortium list: David Adam (retired, Clear Lake Environmental Research Center, Clear Lake, USA), Scott Anderson (School of Earth and Sustainability, Northern Arizona University, Flagstaff, USA), Tripti Bhattacharya (Department of Earth and Environmental Sciences, Syracuse University, Syracuse, USA), Christopher Brierley (Department of Geography, University College London, London, UK), Jordon Bright (School of Earth and Sustainability, Northern Arizona University, Flagstaff, USA), Erik Brown (Department of Earth and Environmental Sciences, University of Minnesota, Duluth, USA), Natalie Burls (Department of Atmospheric, Ocean and Earth Sciences, George Mason University, Fairfax, USA), Kat Cantner (Continental Scientific Drilling Facility, Department of Earth and Environmental Sciences, University of Minnesota, Minneapolis, USA), Leon Clarke (Department of Natural Sciences, Manchester Metropolitan University, Manchester, UK), Timme Donders (Department of Physical Geography, Utrecht University, Utrecht, the Netherlands), Peter Fawcett (Department of Earth and Planetary Sciences, University of New Mexico, Albuquerque, USA), Sarah Feakins (Department of Earth Sciences, University of Southern California, Los Angeles, USA), Troy Ferland (Lamont-Doherty Earth Observatory, Columbia University, Palisades, USA), Sherilyn Fritz (Department of Earth and Atmospheric Sciences and School of Biological Sciences, University of Nebraska, Lincoln, USA), Minmin Fu (Department of Earth and Planetary Sciences, Harvard University, Cambridge, USA), Thomas Giesecke, (Department of Physical Geography, Utrecht University, Utrecht, the Netherlands), Pénelope González-Sampériz (Pyrenean Institute of Ecology (IPE), CSIC, Zaragoza, Spain), Emmanuel Guillerm (Charney School of Marine Sciences, University of Haifa, Haifa, Israel), Matthias Halisch (Petrophysics & Borehole Geophysics Department, Leibniz Institute of Applied Geophysics, Hannover, Germany), Robert Hatfield (Department of Geological Sciences, University of Florida, Gainesville, USA), Timothy Herbert (Department of Earth, Environmental, and Planetary Sciences, Brown University, Providence, USA), Daniel Ibarra (Department of Earth, Environmental, and Planetary Sciences, Brown University, Providence, USA), Emi Ito (Department of Earth and Environmental Sciences, University of Minnesota, Minneapolis, USA), Joseph Janick (independent researcher, Binghamton, New York, USA), Gonzalo Jiménez-Moreno (Department of Stratigraphy and Paleontology, University of Granada, Granada, Spain), Andreas Koutsodendris (Institute of Earth Sciences, Heidelberg University, Heidelberg, Germany), Stephen Kuehn (Department of Physical and Environmental Sciences, Concord University, Athens, USA), Anthony Layzell (Kansas Geological Survey, University of Kansas, Lawrence, USA), Tim Lowenstein (Department of Geological Sciences and Environmental Studies, Binghamton University, Binghamton, USA), Maria Beatrice Magnani (Roy M. Huffington Department of Earth Sciences, Southern Methodist University, Dallas, USA), Miguel Marazuela Calvo (Department of Environmental Geosciences, University of Vienna, Vienna, Austria), David McGee (Department of Earth, Atmospheric, and Planetary Sciences, Massachusetts Institute of Technology, Cambridge, USA), Maud Meijers (Senckenberg Biodiversity and Climate Research Center, Frankfurt, Germany), Steffen Mischke (School of Engineering and Natural Sciences, University of Iceland, Reykjavik, Iceland), Peter Molnar (deceased, Department of Geological Sciences, University of Colorado Boulder, Boulder, USA), Andreas Mulch (Senckenberg–Leibniz Institution for Biodiversity and Earth System Research (SGN), Frankfurt, Germany), Sebastien Nomade (CEA Paris-Saclay and Paris-Saclay University, Gif sur Yvette, France), Anders Noren (Continental Scientific Drilling Facility, Department of Earth and Environmental Sciences, University of Minnesota, Minneapolis, USA), Richard Owen (Department of Geography, Hong Kong Baptist University, Kowloon, Hong Kong), Roberta Pini (Institute of Environmental Geology and Geoengineering, Italian National Research Council, Milano, Italy), Alexander Prokopenko (School of Earth, Ocean & Environment, University of South Carolina, Columbia, USA), Julian Sachs (School of Oceanography, University of Washington, Seattle, USA), James Sample (School of Earth and Sustainability, Northern Arizona University, Flagstaff, USA), Katie Snell (Department of Geological Sciences, University of Colorado Boulder, Boulder, USA), Scott Starratt (United States Geological Survey – Menlo Park, USA), Alison J. Smith (Department of Earth Sciences, Kent State University, Kent, USA), Vania Stefanova (Department of Earth and Environmental Sciences, University of Minnesota, Minneapolis, USA), Camille Thomas (Department of Earth Sciences, University of Geneva, Geneva, Switzerland), Aradhna Tripaati (Department of Atmospheric and Oceanic Sciences and Department of Earth, Planetary, and Space Sciences, University of California, Los Angeles, USA), Blas Valero Garcés (Pyrenean Institute of Ecology (IPE), CSIC, Zaragoza, Spain), Nicholas Waldmann (Dr. Moses Strauss Department of Marine Geosciences, University of Haifa, Haifa, Israel), Jiuyi Wang (Institute of Mineral Resources, Chinese Academy of Geological Sciences, Beijing, PRC), Josef Werne (Department of Geology and Environmental Science, University of Pittsburgh, Pittsburgh, USA), Sarah White (deceased, Department of Geography, University of California, Berkeley, USA), Jack Williams (Department of Geography, University of Wisconsin, Madison, USA), Thomas Wonik (Department of Rock Physics & Borehole Geophysics, Leibniz Institute of Applied Geophysics, Hannover, Germany), Christian Zeeden (Department of Rock Physics & Borehole Geophysics, Leibniz Institute of Applied Geophysics, Hannover, Germany), Yan Zhao (Institute of Geographic Sciences and Natural Resources Research, Chinese Academy of Sciences, Beijing, PRC).
All co-authors contributed to the text and figures that resulted in the submission of a full ICDP proposal and this workshop report. AJS and EI co-chaired the workshop and co-wrote the workshop report with involvement from all co-authors as follows: AJS provided Fig. 1. NB provided Figs. 2 and 3. RH provided Figs. 4 and 5. LC, TD, PM, AK, and BVG revised and edited the final version of the paper. SK, CZ, DM, AP, BVG, and RH provided chronological and stratigraphic input. LC, KS, TL, BVG, and JW provided multi-proxy input. Workshop participants contributed intellectual input.
The contact author has declared that none of the authors has any competing interests.
Publisher’s note: Copernicus Publications remains neutral with regard to jurisdictional claims in published maps and institutional affiliations.
Presentations by Jordan Bright, Natalie Burls, Minmin Fu, Dan Ibarra, Stephen Kuehn, Tim Lowenstein, David McGee, Peter Molnar, Alexander Prokopenko, and Alison J. Smith set the stage for various discussions. Working group verbal summaries were given by Kat Cantner, Timme Donders, Tim Herbert, Tim Lowenstein, Peter Molnar, Anders Noren, Katie Snell, Scott Starratt, Blas Valero Garcés, and Christian Zeeden. We thank Carola Koegler of the ICDP, for logistical help with all three virtual plenary sessions. We report with sadness that two members of the PlioWest Consortium, Peter Molnar and Sarah White, passed away this summer after this paper had been submitted.
This workshop was supported by the International Continental Drilling Program.
This paper was edited by Thomas Wiersberg and reviewed by Julie Brigham-Grette and one anonymous referee.
Allen, R. J. and Luptowitz, R.: El Niño-like teleconnection increases California precipitation in response to warming, Nat. Commun., 8, 1–15, 2017.
Bressler, S. and Butler, R.: Magnetostratigraphy of the Late Tertiary Verde Formation, central Arizona, Earth Planet. Sci. Lett., 38, 319–330, 1978.
Brierley, C. M., Fedorov, A. V., Liu, Z., Herbert, T. D., Lawrence, K. T., and LaRiviere, J. P.: Greatly Expanded Tropical Warm Pool and Weakened Hadley Circulation in the Early Pliocene, Science, 323 1714–1718, https://doi.org/10.1126/science.1167625, 2009.
Burls, N. J. and Fedorov, A. V.: Wetter subtropics in a warmer world: contrasting past and future hydrological cycles, P. Natl. Acad. Sci. USA, 114, 12888–12893, 2017.
Byrne, M. P. and O'Gorman, P. A.: The response of precipitation minus evapotranspiration to climate warming: Why the “wet-get-wetter, dry-get-drier” scaling does not hold over land, J. Climate, 28, 8078–8092, https://doi.org/10.1175/JCLI-D-15-0369.1, 2015.
Chaisson, W. P. and Ravelo, A. C.: Pliocene development of the east-west hydrographic gradient in the tropical Pacific, Paleoceanography, 15, 497–505, 2000.
Colman, S. M., Kaufman, D. S., Bright, J., Heil, C., King, J. W., Dean, W. E., Rosenbaum, J. G., Forester, R. M., Bischoff, J. L., Perkins, M., and McGeehin, J. P.: Age model for a continuous, ca. 250-ka Quaternary lacustrine record from Bear Lake, Utah–Idaho, Quat. Sci. Rev., 25, 2271–2282, 2006.
Dekens, P. S., Ravelo, A. C., and McCarthy, M. D.: Warm upwelling regions in the Pliocene warm period, Paleoceanography, 22, PA3211, https://doi.org/10.1029/2006PA001394, 2007.
Dowsett, H. J., Robinson, M. M., Haywood, A. M., Hill, D. J., Dolan, A. M., Stoll, D. K., Chan, W. L., Abe-Ouchi, A., Chandler, M. A., Rosenbloom, N. A., and Otto-Bliesner, B. L.: Assessing confidence in Pliocene sea surface temperatures to evaluate predictive models, Nat. Clim. Change, 2, 365–371, https://doi.org/10.1038/nclimate1455, 2012.
Fedorov, A. V., Burls, N. J., Lawrence, K. T., and Peterson, L. C.: Tightly linked zonal and meridional sea surface temperature gradients over the past five million years, Nat. Geosci., 8, 975–980, 2015.
Fu, M., Cane, M. A., Molnar, P., and Tziperman, E.: Wetter subtropics lead to reduced Pliocene coastal upwelling, Paleoceanography and Paleoclimatology, 36, e2021PA004243, https://doi.org/10.1029/2021PA004243, 2021.
Fu, M., Cane, M. A., Molnar, P., and Tziperman, E.: Warmer Pliocene upwelling site SST leads to wetter subtropical coastal areas: A positive feedback on SST, Paleoceanography and Paleoclimatology, 37, PA4357, https://doi.org/10.1029/2021PA004357, 2022.
Gardner, J. V., Bradbury, J. P., Dean, W. E., Adam, D. P., and Sarna-Wojcicki, A. M.: Report of Workshop 92 on the correlation of marine and terrestrial records of climate changes in the western United States, 7–10 April 1992, Watsonville, California, USA, US Geological Survey Open-File Report, 92–402, https://pubs.usgs.gov/of/1992/0402/report.pdf (last access: 7 December 2022), 1992.
Groeneveld, J., Steph, S., Tiedemann, R., Garbe-Schonberg, D., Nürnberg, D., and Sturm, A.: Pliocene mixed-layer oceanography for Site 1241, using combined Mg Ca and δ18O analyses of Globigerinoides sacculifer, in: Proc. ODP, Sci. Results, 202, edited by: Tiedemann, R., Mix, A. C., Richter, C., and Ruddiman, W. F., College Station, Texas (Ocean Drilling Program), 1–27, https://doi.org/10.2973/odp.proc.sr.202.209.2006, 2006.
Hansen, J., Sato, M., Russell, G., and Kharecha, P.: Climate sensitivity, sea level and atmospheric carbon dioxide, Philos. T. Roy. Soc. A, 371, 20120294, https://doi.org/10.1098/rsta.2012.0294, 2013.
Hatfield, R. G., Woods, A., Lehmann, S. B., Weidhaas IV, N., Chen, C. Y., Abbott, M. B., and Rodbell, D. T.: Stratigraphic correlation and splice generation for sediments recovered from a large lake drilling project: an example from Lake Junín, Peru, J. Paleolimnol., 63, 83–100, https://doi.org/10.1007/s10933-019-00098-w, 2020.
Held, I. M. and Soden, B. J.: Robust responses of the hydrological cycle to global warming, J. Climate, 19, 5686–5699, https://doi.org/10.1175/JCLI3990.1, 2006.
Herbert, T. D., Lawrence, K. T., Tzanova, A., Peterson, L. C., Caballero-Gill, R., and Kelly, C. S.: Late Miocene global cooling and the rise of modern ecosystems, Nat. Geosci., 9, 843–847, https://doi.org/10.1038/ngeo2813, 2016.
Hildabrand, T. C.: Lithofacies characterization and chemostratigraphy of the Pliocene Verde Formation, northern Verde Valley, Central Arizona, MS Thesis, Northern Arizona University, ProQuest Dissertations & Theses A&I, ProQuest Dissertations & Theses Global, 1710514347, 157 pp., 2015.
House, P. K., Pearthree, P. A., and Perkins, M. E.: Stratigraphic evidence for the role of lake spillover in the inception of the lower Colorado River in southern Nevada and western Arizona, in: Late Cenozoic Drainage History of the Southwestern Great Basin and Lower Colorado River Region: Geologic and Biotic Perspectives, edited by: Reheis, M. C., Hershler, R., and Miller, D. M., Geol. S. Am. S., 439, 335–353, https://doi.org/10.1130/2008.2439(15), 2008.
Ibarra, D. E., Oster, J. L., Winnick, M. J., Rugenstein, J. K. C., Byrne, M. P., and Chamberlain, C. P.: Warm and cold wet states in the western United States during the Pliocene-Pleistocene, Geology, 46, 355–358, 2018.
Lanphere, M. A., Champion, D., Christiansen, R. L., and Obradovich, J. D.: Revised ages for tuffs of the Yellowstone Plateau volcanic field: Assignment of the Huckleberry Ridge Tuff to a new geomagnetic polarity event, Geol. Soc. Am. Bull., 114, 559–568, https://doi.org/10.1130/0016-7606(2002)114<0559:RAFTOT>2.0.CO;2, 2002.
Lanphere, M. A., Champion, D., Clynne, M. A., Lowenstern, J. B., Sarna-Wojcicki, A. M., and Wooden, J. L.: Age of the Rockland tephra, western USA, Quat. Res., 62, 94–10, https://doi.org/10.1016/j.yqres.2004.03.001, 2004.
Lawrence, K. T., Liu, Z.-H., and Herbert, T. D.: Evolution of the eastern tropical Pacific through Plio-Pleistocene glaciation, Science, 312, 79–83, 2006.
Lepar, M. and Ferk, M.: Karst pocket valleys and their implications on Pliocene – Quaternary hydrology and climate: Examples from the Nullarbor Plain, southern Australia, Earth Sci. Rev., 150, 1–13, 2015.
Lisiecki, L. and Raymo, M.: A Pliocene-Pleistocene stack of 57 globally distributed benthic δ18O records, Paleoceanography and Paleoclimatology, 20, PA1003, https://doi.org/10.1029/2004PA001071, 2005.
Lougheed, B. C. and Obrochta, S. P.: A rapid, deterministic age-depth modeling routine for geological sequences with inherent depth uncertainty. Paleoceanography and Paleoclimatology, 34, 122–133, https://doi.org/10.1029/2018PA003457, 2019.
Lukens, W., Fox, D., Snell, K., Wiest, L., Layzell, A., Uno, K., Polissar, P., Martin, R., Fox-Dobbs, K., and Peláez-Campomanes, P: Pliocene paleoenvironments in the Meade Basin, southwest Kansas, USA, J. Sed. Res., 89, 416–439, 2019.
Martin, J. E., Patrick, D., Kihm, A. J., Foit Jr., F. F., and Grandstaff, D. E.: Lithostratigraphy, tephrochronology, and Rare Earth Element geochemistry of fossils at the classical Pleistocene Fossil Lake area, South Central Oregon, J. Geol., 113, 139–155, 2005.
Mass, K. B., Cashman, P. H., and Trexler Jr., J. H.: Stratigraphy and structure of the Neogene Boca basin, northeastern California: Implications for late Cenozoic tectonic evolution of the northern Sierra Nevada, in: Late Cenozoic Structure and Evolution of the Great Basin-Sierra Nevada Transition, edited by: Oldow, J. S. and Cashman, P. H., Geol. S. Am. S., 447, 147–170, https://doi.org/10.1130/2009.2447(09), 2009.
Matthews, N. E., Vazquez, J. A., and Calvert, A. T.: Age of the Lava Creek supereruption and magma chamber assembly at Yellowstone based on 40Ar 39Ar and U-Pb dating of sanidine and zircon crystals, Geochem. Geophy. Geosy., 16, 2508–2528, https://doi.org/10.1002/2015GC005881, 2015.
Menemenlis, S., White, S. M., Ibarra, D. E., and Lora, J. M.: A proxy-model comparison for mid-Pliocene warm period hydroclimate in the Southwestern US, Earth Planet. Sci. Lett., 596, 117803, https://doi.org/10.1016/j.epsl.2022.117803, 2022.
Molnar, P. and Cane, M. A.: El Niño's tropical climate and teleconnections as a blueprint for pre-Ice Age climates, Paleoceanography, 17, 11-1–11-13, https://doi.org/10.1029/2001PA000663, 2002.
Molnar, P. and Cane, M. A.: Early Pliocene (pre-Ice Age) El Niño-like global climate: Which El Niño?, Geosphere, 3, 337–365, 2007.
Moutoux, T. E.: Palynological and tephra correlations among deep wells in the modern Great Salt Lake, Utah, USA: implications for a Neogene through Pleistocene climatic reconstruction, MS Thesis, University of Arizona, 87 pp., https://repository.arizona.edu/handle/10150/191347 (last access: 6 December 2022), 1995.
Nations, J. D., Landye, J. J., and Hevly, R. H.: Location and chronology of Tertiary sedimentary deposits in Arizona: a review, in: Cenozoic Nonmarie Deposits of California and Arizona, Pacific Section, edited by: Ingersoll, R. V. and Woodburne, M. O., SEPM, 107–122, https://archives.datapages.com/data/pac_sepm/037/037001/pdfs/107.htm (last access: 7 December 2022), 1982.
Ogg, J. G.: Geomagnetic polarity time scale, Geologic Time Scale, 1, 159–192, 2020.
Pandya, R. E.: Community driven research in the Anthropocene, in: Future Earth-Advancing Civic Understanding of the Anthropocene, edited by: Dalbotten, D., Roehrig, G., and Hamilton, P., Geophys. Monogr., 203, American Geophysical Union, Washington, D.C., 203, 53–66, ISBN 978-1-118-85430-3, 2014.
Ravelo, A. C., Dekens, P. S., and McCarthy, M.: Evidence for El Niño–like conditions during the Pliocene, GSA Today, 16, 4–11, 2006.
Rieck, H. J., Sarna-Wojcicki, A. M., Meyer, C. E., and Adam, D. P.: Magnetostratigraphy and tephrochronology of an upper Pliocene to Holocene record in lake sediments at Tulelake, northern California, Geol. Soc. Am. Bull., 104, 409–428, 1992.
Rivera, T. A., Mark, D., Schmitz, M. D., Jicha, B. R., and Crowley, J. L.: Zircon petrochronology and 40Ar 39Ar sanidine dates for the Mesa Falls Tuff: crystal-scale records of magmatic evolution and the short lifespan of a large Yellowstone magma chamber, J. Petrol., 57, 1677–1704, https://doi.org/10.1093/petrology/egw053, 2016.
Rundel, P. W., Arroyo, M. T., Cowling, R. M., Keeley, J. E., Lamont, B. B., and Vargas, P.: Mediterranean biomes: evolution of their vegetation, floras, and climate, Annu. Rev. Ecol. Evol. S., 47, 383–407, 2016.
Ryan, W. B. F., Carbotte, S. M., Coplan, J., O'Hara, S., Melkonian, A., Arko, R., Weissel, R. A., Ferrini, V., Goodwillie, A., Nitsche, F., Bonczkowski, J., and Zemsky, R.: 2009, Global Multi-Resolution Topography (GMRT) synthesis data set, Geochem. Geophy. Geosy., 10, Q03014, https://doi.org/10.1029/2008GC002332, 2009.
Sarna-Wojcicki, A. M., Morrison, S. D., Meyer, C. E., and Hillhouse, J. W.: Correlation of upper Cenozoic tephra layers between sediments of the western United States and eastern Pacific Ocean and comparison with biostratigraphic and magnetostratigraphic age data, Geol. Soc. Am. Bull., 98, 207–223, 1987.
Sarna-Wojcicki, A. M., Meyer, C. E., Adam, D. P., and Sims, J. D.: Correlations and age estimates of ash beds in late Quaternary sediments of Clear Lake, California, in: Late Quaternary Climate, Tectonism and Sedimentation in Clear Lake, Northern California Coast Ranges, edited by: Sims, J. D., Geol. S. Am. S., 214, 141–150, 1988.
Sarna-Wojcicki, A. M., Deino, A. L., Fleck, R. J., McLaughlin, R. J., Wagner, D., Wan, E., Wahl, D., Hillhouse, J. W., and Perkins, M.: Age, composition, and areal distribution of the Pliocene Lawlor Tuff, and three younger Pliocene tuffs, California and Nevada, Geosphere, 7, 599–628, https://doi.org/10.1130/GES00609.1, 2011.
Schonher, T. and Nicholson, S. E: The Relationship between California Rainfall and ENSO Events, J. Climate, 2, 1258–1269, 1989.
Seager, R. and Vecchi, G. A.: Greenhouse warming and the 21st century hydroclimate of southwestern North America, P. Natl. Acad. Sci. USA, 107, 21277–21282, https://doi.org/10.1073/pnas.0910856107, 2010.
Seager, R., Naik, N., Ting, M., Cane, M. A., Harnik, N., and Kushnir, Y.: Adjustment of the atmospheric circulation to tropical Pacific SST anomalies: Variability of transient eddy propagation in the Pacific-North America sector, Q. J. Roy. Meteor. Soc., 136, 277–296, 2010.
Smith, G. I., Barczak, V. J., Moulton, G., and Liddicoat, J. C.: Core KM-3, a surface-to-bedrock record of late Cenozoic sedimentation in Searles Valley, California. US Geol Surv Prof P, 1256, U.S. Government Printing Office, Washington, D.C., 1–24, https://doi.org/10.3133/pp1256, 1983.
Stap, L. B., de Boer, B., Ziegler, M., Bintanja, R., Lourens, L. J., and van de Wal, R. S. W.: CO2 over the past 5 million years: Continuous simulation and new δ11B-based proxy data, Earth Planet. Sci. Lett., 439, 1–10, 2016.
Thompson, R. S.: Pliocene and early Pleistocene environments and climates of the western Snake River Plain, Idaho, Mar. Micropaleontol., 27, 141–156, 1996.
Tierney, J. E., Haywood, A. M., Feng, R., Bhattacharya, T., and Otto-Bliesner, B. L.: Pliocene warmth consistent with greenhouse gas forcing, Geophys. Res. Lett., 46, 9136–9144, 2019.
Trenberth, K. E., Branstator, G. W., Karoly, D., Kumar, A., Lau, N.-C., and Ropelewski, C.: Progress during TOGA in understanding and modeling global teleconnections associated with tropical sea surface temperatures, J. Geophys. Res.-Atmos., 103, 14291–14324, 1998.
Wara, M. W., Ravelo, A. C., and Delaney, M. L.: Permanent El Niño-like conditions during the Pliocene Warm Period, Science, 309, 758–761, https://doi.org/10.1126/science.1112596, 2005.
Williams, S. K.: Late Cenozoic tephrostratigraphy of deep sediment cores from the Bonneville Basin, northwest Utah, Geol. Soc. Am. Bull., 106, 1517–1530, 1994.
Winnick, M. J., Welker, J. M., and Chamberlain, C. P.: Stable isotopic evidence of El Niño-like atmospheric circulation in the Pliocene western United States, Clim. Past, 9, 903–912, https://doi.org/10.5194/cp-9-903-2013, 2013.
Zeeden, C., Rivera, T. A., and Storey, M.: An astronomical age for the Bishop Tuff and concordance with radioisotopic dates, Geophys. Res. Lett., 41, 3478–3484, https://doi.org/10.1002/2014GL059899, 2014.
Zurba, M., Petriello, M. A., Madge, C., McCarney, P., Bishop, B., McBeth, S., Denniston, M., Bodwitch, H., and Bailey, M.: Learning from knowledge co-production research and practice in the twenty-first century: global lessons and what they mean for collaborative research in Nunatsiavut, Sustain. Sci., 17, 449–467, https://doi.org/10.1007/s11625-021-00996-x, 2022.