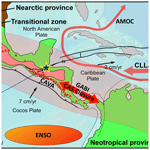
Planning for the Lake Izabal Basin Research Endeavor (LIBRE) continental scientific drilling project in eastern Guatemala
Andreas Eckert
Peter M. J. Douglas
Liseth Perez
Alex Correa-Metrio
Bronwen L. Konecky
Thorsten Bauersachs
Susan Zimmerman
Stephanie Scheidt
Mark Brenner
Steffen Kutterolf
Jeremy Maurer
Omar Flores
Caroline M. Burberry
Anders Noren
Amy Myrbo
Matthew Lachniet
Nigel Wattrus
Derek Gibson
As Earth's atmospheric temperatures and human populations increase, more people are becoming vulnerable to natural and human-induced disasters. This is particularly true in Central America, where the growing human population is experiencing climate extremes (droughts and floods), and the region is susceptible to geological hazards, such as earthquakes and volcanic eruptions, and environmental deterioration in many forms (soil erosion, lake eutrophication, heavy metal contamination, etc.). Instrumental and historical data from the region are insufficient to understand and document past hazards, a necessary first step for mitigating future risks. Long, continuous, well-resolved geological records can, however, provide a window into past climate and environmental changes that can be used to better predict future conditions in the region. The Lake Izabal Basin (LIB), in eastern Guatemala, contains the longest known continental records of tectonics, climate, and environmental change in the northern Neotropics. The basin is a pull-apart depression that developed along the North American and Caribbean plate boundary ∼ 12 Myr ago and contains > 4 km of sediment. The sedimentological archive in the LIB records the interplay among several Earth System processes. Consequently, exploration of sediments in the basin can provide key information concerning: (1) tectonic deformation and earthquake history along the plate boundary; (2) the timing and causes of volcanism from the Central American Volcanic Arc; and (3) hydroclimatic, ecologic, and geomicrobiological responses to different climate and environmental states. To evaluate the LIB as a potential site for scientific drilling, 65 scientists from 13 countries and 33 institutions met in Antigua, Guatemala, in August 2022 under the auspices of the International Continental Scientific Drilling Program (ICDP) and the US National Science Foundation (NSF). Several working groups developed scientific questions and overarching hypotheses that could be addressed by drilling the LIB and identified optimal coring sites and instrumentation needed to achieve the project goals. The group also discussed logistical challenges and outreach opportunities. The project is not only an outstanding opportunity to improve our scientific understanding of seismotectonic, volcanic, paleoclimatic, paleoecologic, and paleobiologic processes that operate in the tropics of Central America, but it is also an opportunity to improve understanding of multiple geological hazards and communicate that knowledge to help increase the resilience of at-risk Central American communities.
- Article
(15139 KB) - Full-text XML
- BibTeX
- EndNote
Drilling and coring in continental settings has provided answers to compelling scientific questions and has enabled us to better understand Earth and forecast how Earth Systems might behave in the future (Colman, 1996; Harms et al., 2007; NRC, 2011; Soreghan and Cohen, 2013; NASEM, 2020). Long sediment records have allowed us to infer tectonic, volcanic, climatic, environmental, and ecological processes on long temporal scales (Soreghan and Cohen, 2013). However, we still lack records from key regions around the world, including continental Central America. Despite being a relatively small region, Central America has played an outsized role in influencing several Earth System processes through geologic time (Fig. 1). For example, a major continental strike-slip fault system in Guatemala forms part of the North American–Caribbean plate boundary (Lyon-Caen et al., 2006; Authemayou et al., 2012; Ellis et al., 2019; Guzmán-Speziale and Molina, 2022) and has caused devastating earthquakes in the past (Plafker, 1976; White, 1984, 1985). The area also possesses one of the most active volcanic chains in the world along the Pacific coast (Kutterolf et al., 2008a) and hosts important biological hotspots (Graham, 2010; Correa-Metrio et al., 2011). Central American mountains influence easterly winds that affect the El Niño–Southern Oscillation and regional precipitation patterns (Baldwin et al., 2021). The closure of the Central American Isthmus that connects North and South America caused one of the most important biotic interchanges in recent geologic history (Bacon et al., 2015), modified global ocean circulation patterns (Sentman et al., 2018), and may have helped establish Northern Hemisphere glaciation (Haug et al., 2001; cf. Molnar, 2008). The many geological processes that affect the region expose a large proportion of the Central American population to multiple hazards, some of which are not well characterized and are poorly understood.
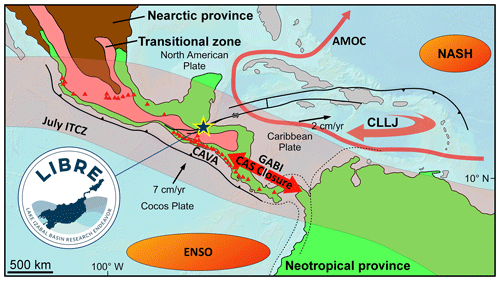
Figure 1Tectonic map of Central America and northern South America (modified from Mann, 2007) showing the diverse Earth System processes that interact in the vicinity of the Lake Izabal Basin (yellow star). The basin is located at the boundary between the North American and Caribbean tectonic plates, where devastating earthquakes have occurred in the past. The basin is also located at the transitional zone between Nearctic and Neotropical provinces (Holt et al., 2013). Several ocean–atmosphere processes influence regional hydroclimate, such as the position of the Intertropical Convergence Zone (ITCZ), the El Niño–Southern Oscillation (ENSO), the North Atlantic Subtropical High (NASH), and the Caribbean Low-Level Jet (CLLJ). Closure of the Central American Seaway (CAS closure) resulted in the Great American Biotic Interchange (GABI) and in changes in the Atlantic Meridional Overturning Circulation (AMOC). Finally, the basin is located north of one of the most active volcanic chains in the world, the Central American Volcanic Arc (CAVA).
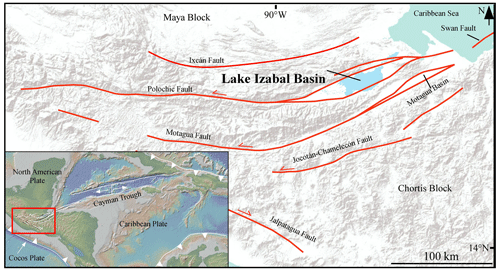
Figure 2Topographic and tectonic map of southern Guatemala showing the main fault traces of the Polochic–Motagua Fault System and the location of the Lake Izabal Basin. Inset map shows the tectonic and topographic map of the Caribbean region, highlighting the location of the study area and the North American and Caribbean plate boundary. Modified from Obrist-Farner et al. (2020).
The Lake Izabal Basin (LIB) in eastern Guatemala is an active pull-apart basin along the North American and Caribbean plate boundary (Figs. 1, 2), with > 4 km of sediment that likely began to accumulate during the Miocene (∼ 12 Myr ago; Obrist-Farner et al., 2020). Seismic stratigraphic investigations indicate that the basin is deep and asymmetric: it is the result of a complex interplay between tectonics and sedimentation (Bartole et al., 2019). The accumulated deposits preserve potentially unrivalled continental paleoclimatic, volcanic, paleoseismic, and paleoecologic records of the region. The location of the basin along a major tectonic plate boundary, the thick and asymmetric sediment record, and frequent eruption of tephras for chronology make the LIB an ideal location for a project within the International Continental Scientific Drilling Program (ICDP). Drilling in the LIB will enable better quantification of seismic hazards and coring the basin's asymmetric infill will provide a continuous Miocene-to-recent sediment record. Recovery of such a long record will provide opportunities to investigate the following: (1) the seismo-tectonic evolution of the basin and of the North American and Caribbean plate boundary; (2) neotropical hydroclimate from the late Miocene to present, providing constraints on terrestrial hydroclimate responses to tectonic, greenhouse gas, and orbital variations; (3) biological and ecological dynamics related to the period during which the land bridge developed between North and South America and related to glacial–interglacial cycles that were established in the Quaternary; (4) the history of explosive volcanism from the northern Central American Volcanic Arc (CAVA); and (5) the diversity and abundance of life in the terrestrial deep biosphere, as well as feedback dynamics on nutrient cycles (e.g., carbon and nitrogen) through geomicrobiological processes.
In August 2022, we organized and held a workshop in the city of Antigua, Guatemala, with 65 scientists from 13 countries and 33 institutions. Workshop discussions focused on the rationale for the project and drafting of scientific questions and hypotheses that can be addressed using instrumented boreholes and drill cores from the basin, and chronological tools and outreach activities. With that in mind, the group identified drilling targets and discussed logistics associated with a drilling campaign. Key government and industry collaborators in Guatemala donated data sets that were discussed during the workshop. For instance, the Guatemalan government provided seismic data from the LIB that was acquired by the Arco oil company in 1978 (Fig. 3), of which 332 km was acquired in Lake Izabal and 127 km in the alluvial plain to the east. The Shell Oil Company reprocessed all Arco lines in 1991 and acquired an additional 69 km of seismic lines on land and 431 km in Lake Izabal. In addition, the Guatemalan government made available all data from the Colorado-1 well, which was drilled in 1993 on the eastern side of the LIB (Fig. 3) and provides information related to lithologies, seismic velocities, and age of the basin fill. The data sets are key to our understanding of the LIB and helped us formulate hypotheses based on the potential geological time intervals we expect to recover from the basin.
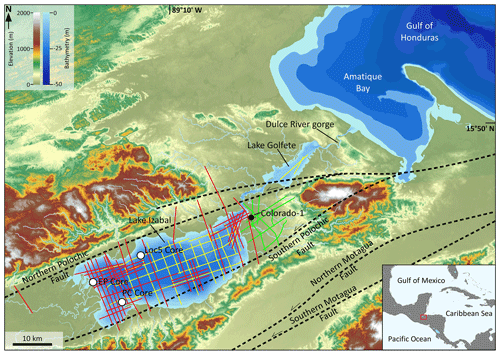
Figure 3Topographic and bathymetric map of the Izabal region. White circles show existing coring locations discussed in the text. Yellow and green lines show the location of the 1978 Arco seismic lines, and red lines show the location of the 1991–1992 Shell seismic lines. Major faults are shown with thick dashed black lines. Inset shows a map of Central America and part of the Caribbean, with the location of the Izabal region in eastern Guatemala indicated by the red box.
During the workshop, participants discussed available data from the LIB. The LIB is 80 km long and 20 km wide (Fig. 2). The eastern side of the basin is occupied by Lake Izabal (15∘30′ N, 89∘10′ W), whereas the western side contains a wetland of international importance, the Ramsar site Bocas del Polochic (Ramsar site no. 813). Lake Izabal is shallow (zmax = 15 m) and polymictic, with a temperature difference between surface and bottom waters of only ∼ 2 ∘C (Brinson and Nordlie, 1975). Mean annual precipitation in the region is ∼ 3300 mm (Duarte et al., 2021), and the climate is characterized by a rainy season that extends from May through December and a dry season from January to April (Duarte et al., 2021). The basin is confined to the north by the Santa Cruz Mountain Range, to the south by the Minas Mountain Range, and to the east by the Mico Mountains (Fig. 3).
Outcrop studies, seismic stratigraphic analysis, and syntheses of industry well data provide constraints on the timing of basin formation and the basin's stratigraphic architecture. Three formations make up the Tertiary stratigraphy of the Izabal region (Fig. 4; Powers, 1918; Vinson, 1962; Mota-Vidaure, 1989; Obrist-Farner et al., 2020). The oldest formation is the Upper Oligocene to Lower Miocene Rio Dulce Formation, which rests unconformably on top of marine Permian limestones (Vinson, 1962). The Rio Dulce Formation consists of marine limestones that are light buff, tan, and cream-colored, with abundant coral and mollusk fragments (Powers, 1918; Vaughan, 1919; Vinson, 1962). The formation is overlain unconformably by the informally defined terrigenous mid-Miocene Carboneras formation (Mota-Vidaure, 1989). The lower part of the Carboneras formation is constrained to the Serravallian by zircon weighted-mean 206Pb 238U ages of 12.060 ± 0.008 Ma from a volcanic tuff ∼ 30 m above the Rio Dulce–Carboneras unconformity (Obrist-Farner et al., 2020). The Carboneras formation is composed of claystone, siltstone, marl, sandstone, conglomerate, and lignite beds (Mota-Vidaure, 1989; Obrist-Farner et al., 2020). The uppermost formation is the Herrería Formation, constrained to the Pliocene–Pleistocene based on freshwater gastropod shells (Powers, 1918). The formation is composed of claystone, siltstone, marl, sandstone, conglomerate, and lignite beds (Powers, 1918; Vinson, 1962). Correlation between outcrop information and seismic profiles by Obrist-Farner et al. (2020) indicates that the Carboneras formation forms part of the initial infill of the LIB, constraining basin initiation to the mid-Miocene.
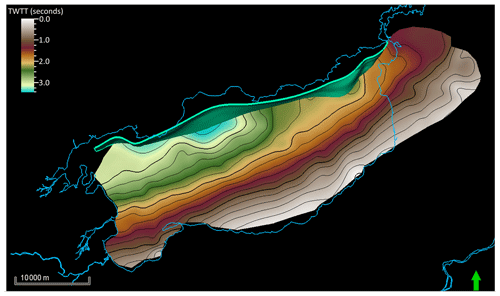
Figure 5Basement contour map in two-way travel time (TWTT) of the Lake Izabal Basin (LIB). Green fault is the main principal deformation zone of the LIB. On the eastern side of the basin, the basement has been uplifted and is closer to the surface than it is on the western side, an area where more than 4 km of sediment has accumulated.
Seismic stratigraphic studies revealed that the basin is asymmetric in both a north–south and an east–west direction. In a north–south direction, the basin geometry resembles that of a half-graben, resulting from transform-normal extension along the Polochic Fault (Ben-Avraham and Zoback, 1992), with the thickest sedimentary package occurring parallel to the principal deformation zone (Bartole et al., 2019: Fig. 5). In an east–west direction, basin asymmetry resulted from a migrating depocenter similar in form to extant (Crowell, 2003) and young (Beeson et al., 2017) pull-apart basins along the San Andreas Fault, California (USA). The LIB depocenter has migrated ∼ 70 km during Polochic Fault displacement (Bartole et al., 2019), with a basin infill that thickens on the western side (Fig. 5). Constraints on the basin stratigraphy are based on the Colorado-1 industry well (Shell Oil Company, Exploradora y Productora de Guatemala: Informe final de exploración, unpublished report, 1993). Located on the eastern side of the basin (Fig. 3), the well was drilled through 1400 m of sediment before it was plugged and abandoned. Cuttings from the well indicate that the lowermost strata (1400–500 m) are composed of mudstone with thin sandstone beds, with the appearance of coal from 1000 to 800 m and conglomerate from 600 to 500 m. Conglomerate and sandstone content become more dominant from 500 to ∼ 200 m, with an increase in mudstone and limestone from 200 m to the surface (Obrist-Farner et al., 2020). Correlation among outcrops, seismic profiles, and the Colorado-1 well indicate that the 1400 m drilled by the well is younger than 12 Ma (Obrist-Farner et al., 2020), and preliminary palynological results support this interpretation based on Tortonian-age pollen (∼ 8 Ma) found in cutting samples at ∼ 1200 m.
Short sediment cores from Lake Izabal further constrain the stratigraphy of the basin and reveal climate and environmental change during the Holocene. Variations in elemental abundances in a 7.6 m radiocarbon-dated sediment core obtained from the northwest side of the basin (Loc5 in Fig. 3) suggest a hydroclimate shift from drier to wetter conditions from the early to the mid-Holocene and stable hydroclimatic conditions thereafter until ∼ 1000 years ago when drier conditions returned (Duarte et al., 2021). Superimposed on this long-term trend is a marine incursion during the 8.2 ka event (Obrist-Farner et al., 2022), highlighting the sensitivity of this low-lying lake to sea-level variations. This marine incursion resulted in an ecosystem shift, modifying the low-lying lake for ∼ 4500 years. A shorter sediment core from the southern side of the basin (PC in Fig. 3) spans the last 1400 years, revealing interesting hydroclimate changes during the Little Ice Age (LIA). In contrast to many records from Central America that suggest a drier hydroclimate during the LIA, evidence from Izabal suggests increased runoff (Obrist-Farner et al., 2023) and increased tropical forest cover after substantial human-mediated deforestation during ancient Maya times, which ended ca. 1000 years ago (Mongol et al., 2023). In addition, a shorter sediment core obtained near the Polochic Delta (EP core in Fig. 3) revealed several geochemical variations that indicate human disturbance of Lake Izabal during the last 100 years, including an increase in the lake's trophic status (Obrist-Farner et al., 2019) and metal contamination caused by mining activities in the catchment (Hernández et al., 2020). Together, these data sets indicate that the sedimentary archive of the LIB contains records of paleoseismic, paleoclimatic, and paleoenvironmental changes across various timescales.
The LIBRE project is well positioned to address two science priorities identified by ICDP (Anselmetti et al., 2020), using an integrated scientific approach, as follows: (1) understanding the full chain from geologic hazard to risk (Theme 02: geohazards), and (2) exploring sedimentary archives to understand Earth's evolution (Theme 04; environmental change). Expanding on these two themes, the LIBRE workshop focused on defining scientific priorities and questions, logistics, drilling targets, and education and outreach plans. The resulting discussions were synthesized into five scientific motivations and an outreach and education plan.
4.1 Tectonic processes and seismic risk (ICDP Theme 02)
The LIB is an ideal scientific drilling site because it is a pull-apart basin that developed along the Polochic–Motagua Fault System (PMFS; Lodolo et al., 2009), a system comprised of four sub-parallel faults that separate the North American and Caribbean plates in Guatemala (Figs. 1, 2; Lyon-Caen et al., 2006). Through geologic time, basin depocenter migration and continued subsidence played a large role in the morphology of the basin. For example, the depocenter migrated ∼ 70 km at the speed of the transform (Bartole et al., 2019), recording the complex interplay between tectonic deformation and sedimentation. The area is also subject to intense seismic activity, as evidenced by the 1976 Motagua earthquake (Plafker, 1976) and by estimates of destruction and shaking during the estimated Mw 7.6 Polochic earthquake in 1816 (White, 1985). In addition, high-resolution geophysical surveys of Lake Izabal's floor revealed contemporary surface fractures (Fig. 6). Despite evidence of contemporary deformation and potential for catastrophic earthquakes, knowledge of the tectonic and displacement history of the main faults and their associated seismic hazard are largely unknown and poorly characterized. A continental scientific drilling project in the LIB, involving long sediment cores and a borehole-based observatory (Sect. 5), will bridge tectonic timescales and enable us to: (1) constrain paleoseismic events and test whether earthquakes along the plate boundary occur in a random, quasi-periodic, or clustered manner; (2) estimate the speed of the transform by providing age constraints for the basin's stratigraphy (e.g., Beeson et al., 2017); and (3) monitor and record earthquake processes in very close proximity to active faults. Such information is crucial to test hypotheses related to the tectonic role, seismicity, and seismic risk of the Polochic Fault and help investigate how fault networks interact via propagation, abandonment, and reactivation across a range of spatial and temporal scales. These field observations are also needed to improve knowledge of seismic hazards and develop numerical models aimed at understanding how fault networks evolve through time and how seismic hazard is distributed (Huntington and Klepeis, 2018; Tsai et al., 2021; Ben-Zion et al., 2022).
4.2 A rich volcanic archive (ICDP Theme 02)
Tephra deposition in Central America is controlled by one of the most active volcanic arcs in the world, which has been responsible for several devastating Plinian and ignimbrite-forming eruptions (Kutterolf et al., 2008a). Marine sediment cores (∼ 10 m long) from the Pacific Ocean revealed a tephra inventory of more than 400 individual layers that provide evidence of > 100 eruptions spanning the last ∼ 400 kyr (Drexler et al., 1980; Kutterolf et al., 2008a, b, 2016). Long Deep Sea Drilling Project (DSDP) and Ocean Drilling Program (ODP) cores from 14 sites offshore of Guatemala and El Salvador revealed single eruptive events from CAVA, dating back to the Miocene (Schindlbeck et al., 2018). Eruptive events are also evident in sediment records from the Caribbean Sea, which recorded Miocene ignimbrite-forming eruptions and explosive volcanic events from Central America (Sigurdsson et al., 2000; Jordan et al., 2007). Highly resolved, long sediment cores from the LIB can thus provide long-term Neogene and Quaternary information to test hypotheses related to the systematic changes in both the frequency of volcanic activity and compositional variations through time and possible external controls on CAVA activity – information needed to better understand volcanic hazards in the region.
4.3 Paleoclimatic reorganizations and analogs for modern climate change (ICDP Theme 04)
The sediments of the LIB record the response of terrestrial climate to tectonic, greenhouse gas, and orbital variations over the last ∼ 12 Myr, providing a unique opportunity to investigate a hydroclimate record from the Neotropics that extends back to the late Miocene. A continental drilling project in the LIB will enable the study of climate evolution during the late Miocene, Pliocene, and through the Quaternary.
Expansion of C4 plants in regions such as North America, tropical Africa, and Indo-Asia during the late Miocene and Pliocene points to major ecosystem shifts (Strömberg and McInerney, 2011; Uno et al., 2011; Feakins et al., 2020). However, the expansion appears to have been region-specific (Strömberg, 2011) and likely the result of increased overall aridity and changes in the seasonality of precipitation (Pagani et al., 1999; Strömberg and McInerney, 2011). However, only sparse late Miocene to Pliocene paleoclimate data exist from the Neotropics to determine the regional expression of these changes. In addition, the paucity of long Central American paleoclimate records makes it difficult to resolve debates about the drivers of tropical climate change during the mid-Pliocene, a time of higher atmospheric CO2 and global warmth, and a prospective analog for present-day climate change (Burke et al., 2018).
Superimposed on long-term cooling, closure of the Central American Seaway (CAS) spanned ∼ 7 to 4 Myr, with the last near-surface ocean connection closing by ca. 2.5 Ma (O'Dea et al., 2016; McGirr et al., 2020). CAS closure caused profound changes in the Atlantic Ocean, with a strengthening of the Atlantic Meridional Overturning Circulation (AMOC; Haug and Tiedemann, 1998; Auderset et al., 2019). Impacts of CAS closure on global climate are debated (Utescher et al., 2017 vs. Molnar, 2008) and model simulations suggest that southern Central America and northern South America became generally drier and colder (Fedorov et al., 2013, 2015; Brierley and Fedorov, 2016). On glacial and orbital timescales, the LIB offers an opportunity to extend paleoclimate records through the entire Quaternary; i.e., the past ∼ 2.6 Myr. The current lack of continuous Quaternary records precludes the evaluation of climate drivers during key time intervals, such as before and after the Mid-Pleistocene Transition (MPT) ∼ 1.25–0.70 Ma, when global ice age cycles intensified and moved from 41 to 100 ka pacing (Chalk et al., 2017). Therefore, a long, continuous, and high-resolution record from the LIB will provide an unrivalled opportunity to reconstruct neotropical climate from the late Miocene to the present, enabling testing of hypotheses related to precipitation changes as a result of CAS closure, potential drying caused by reduced convection during the mid-Pliocene, and changes in hydroclimate as a result of the establishment of Northern Hemisphere glaciation.
4.4 Paleoecological evolution and the American biotic exchange (ICDP Theme 04)
The LIBRE project offers the opportunity to explore the composition and structure of neotropical biological communities from the Miocene to present. Closure of the CAS marked one of the most important events in terms of the biogeography of the Neotropics (Jaramillo, 2018). Although species interchange between the Nearctic and Neotropical biogeographic realms started before the Miocene (Bacon et al., 2015), closure of the CAS accelerated migration rates, triggering ecological dynamics of community rearrangement. Before the complete emergence of the CAS, northern South America and southern Central America were mostly occupied by rainforests and mangroves, under a warm and wet climate regime (Graham, 1998; Salzmann et al., 2008; Jaramillo et al., 2020). A long sediment record from the LIB could help constrain how climate change after CAS closure modified the availability of environmental niches in the Neotropics, leading to the synthesis of novel ecosystems in the region (Correa-Metrio et al., 2012; Pérez et al., 2021).
Furthermore, a Quaternary record from the LIB can contribute to our understanding of how regional vegetation changed in response to glacial–interglacial dynamics. Palynological data suggest that during the Quaternary, vegetation changes in the northern Neotropics were characterized by relatively sparse forest cover, dominated by Nearctic elements during glacials and dense Neotropical forests during interglacials (Correa-Metrio et al., 2012). Thus, after the establishment of Northern Hemisphere glaciation, vegetation changes were likely associated with the formation/disappearance of corridors that promoted/impeded migration (Bacon et al., 2016). Differing migration patterns, together with climate variability, probably resulted in the synthesis of novel and “no-modern-analog” ecosystems in the region (Correa-Metrio et al., 2012; Perez et al., 2021). Long Quaternary records from the Izabal Basin will also enable us to understand vegetation changes in what is one of the currently wettest areas of Central America, and one that is influenced by climate drivers different from those that affect sites in the drier northeast lowlands of Central America and Mexico. For instance, the Lake Izabal region is thought to have provided a haven for biodiversity (refugia) during past periods of climate change (Prance, 1982), and long sediment cores from the Quaternary will allow us to investigate the role of the region in maintaining regional ecosystems and biomes during times of climatic turmoil. Furthermore, we will be able to investigate relationships between the structure of aquatic and terrestrial ecosystems and sea-level variability (Obrist-Farner et al., 2022).
4.5 Life in the deep biosphere: a continental lacustrine view (ICDP Theme 04)
The deep biosphere, microbial life that thrives in sediments down to several kilometers of depth, plays a major role in global biogeochemical cycles of carbon, nitrogen, and sulfur. Our knowledge of the community composition and abundance of the deep biosphere relies largely on studies carried out within the framework of the Integrated Ocean Discovery Program (IODP) and its predecessors over the past 3 decades (Parkes et al., 1994; D'Hondt et al., 2004). Such studies have provided a wealth of information on the nature and abundance of microbial life, showing that life can exist to ∼ 10 km below the surface and at temperatures that may reach > 120 ∘C (Inagaki et al., 2015; Heuer et al., 2020). They have also demonstrated that the genetic diversity of the deep biosphere and its abundance rivals that found in the overlying ocean waters (Kallmeyer et al., 2012), but data from continental settings are largely missing. In general, our understanding of the composition, abundance, and evolution of life in deeply buried lacustrine archives is sparse, hindering an understanding of the role of the continental deep biosphere in global biogeochemical cycles. With a Miocene-to-recent sediment record, Lake Izabal offers a unique opportunity to study the community composition of the continental deep biosphere at an unprecedented scale and resolution. It will also provide key insights into the vertical zonation and abundance of microbial cells. Given the generally higher productivity and organic matter preservation of lake systems compared to the marine realm, we expect that deeply buried lacustrine sediment sequences are oases of life and hot spots of microbial activity on the continents, but such deposits remain terra incognita. Furthermore, the sensitivity of Lake Izabal to sea-level variability (Obrist-Farner et al., 2022) offers an opportunity to understand how repeated marine incursions into Lake Izabal may have influenced the development of hot spots of microbial life in the deeply buried sediments.
4.6 Increasing public engagement with geology in Guatemala
An important component of the LIBRE project is its education and outreach plan. During the workshop, participants focused on several ideas to build public interest in the drilling project. These include: (1) involvement of Guatemalan scientists who have been working in Lake Izabal and on seismic hazard assessment in the country; (2) participation of Guatemalan geoscience undergraduate and graduate students; (3) collaboration with the Authority for the Sustainable Management of Lake Izabal (AMASURLI), the NGO Defensores de la Naturaleza, which is in charge of protecting the Izabal wetland, and the Guatemalan Institute for Seismology, Volcanology, Meteorology, and Hydrology (INSIVUMEH); (4) development of Earth Science stations at museums in Guatemala City and in the offices of AMASURLI and Defensores de la Naturaleza, near Lake Izabal; and (5) production of a book targeted to 10–13-year-old children that highlights the spectacular geology and environments of Guatemala, with the main focus related to the ICDP drilling project.
The LIBRE workshop participants discussed drilling locations and operations within the framework of the scientific questions and generated specific drilling targets and borehole instrumentation plans. A consensus was reached on establishing a comprehensive three-component drilling plan that involves a network of instrumented boreholes and collection of long sediment cores, both on land and in Lake Izabal. These include a deep master borehole cored and instrumented onshore on the eastern side of the LIB, six instrumented seismic observatory onshore boreholes in the vicinity of Lake Izabal, and two coring sites offshore in Lake Izabal (Fig. 7). All drilling locations were selected and identified based on: (1) seismic data from the LIB; (2) stratigraphic correlations between seismic lines and the reconstructed progradational infill of the basin (Bartole et al., 2019), ensuring stratigraphic overlap between coring sites and complete recovery of the sediment record to 8 Ma; (3) the velocity model and preliminary palynological work for the Colorado-1 well; and (4) the geologic map (Bonis et al., 1970) and revised stratigraphy for the Izabal region (Obrist-Farner et al., 2020; Fig. 4).
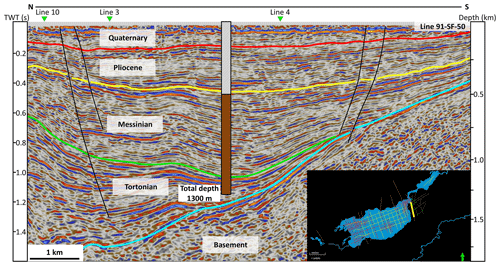
Figure 8Interpreted seismic line 91-SF-50 showing the location and total depth of the master borehole (brown) and the proposed age of the sediment package. The inset map shows the location of profile 91-SF-50 on the eastern side of the Lake Izabal Basin.
We discussed the drilling and coring of a master borehole on the alluvial plain along the eastern side of the LIB. The area is along seismic line 91-SF-50, 4 km from the location of the Colorado-1 well (Fig. 8) and near two crossing lines (lines 3 and 4; Figs. 7, 8). The master borehole will drill through 500 m of sediment without core recovery because this interval is most likely composed of sandstone and conglomerate, based on information from the Colorado-1 well. We discussed the possibility of then collecting a core from 500 to 1300 m, avoiding drilling deeper because of logistical challenges, available technology, and associated costs. Seismic interpretation (Fig. 8), in combination with the velocity model from the Colorado-1 well and preliminary palynological results, indicates that the 800 m core will contain thick mudstone intervals with minimal sandstone and will at least contain sediment from the Tortonian (∼ 8 Ma) to the Messinian and Pliocene (∼ 5 Ma). The borehole will then be used as a seismic observatory, with the installation of seismometers and strainmeters at multiple depths.
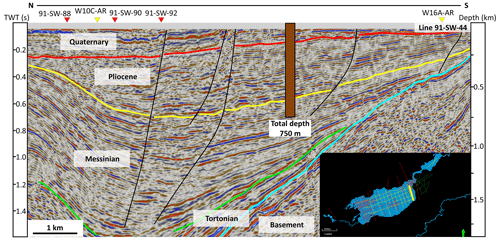
Figure 9Interpreted seismic line 91-SW-44 showing the location and total depth of the eastern lake core (brown) and the proposed age of the sediment package. The inset map shows the location of profile 91-SW-44 on the eastern side of the Lake Izabal Basin.
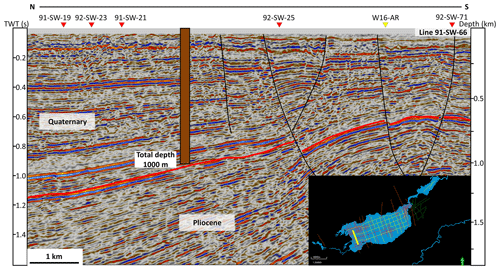
Figure 10Interpreted seismic line 91-SW-66 showing the location and total depth of the eastern lake core (brown) and the proposed age of the sediment package. The inset map shows the location of profile 91-SW-66 on the western side of the Lake Izabal Basin.
We identified two coring locations within Lake Izabal. The first site is in the eastern side of the lake, located along inline 91-SW-44 and near crossing line 91-SW-92 (Figs. 7, 9). The location is ∼ 5 km from the master borehole site and is in an area where the lake is 15 m deep. A core will be collected to a depth of ∼ 750 m and based on seismic interpretation will contain sediments deposited from the Messinian (∼ 6 Ma) to the present. The second site is on the western side of Lake Izabal, located along inline 91-SW-66 and crossline 91-SW-21 (Figs. 7, 10). We plan to collect a 1000 m core from this site. Seismic interpretation indicates that the 1000 m core will contain a record from ∼ 2.5 Ma to the present, providing a highly resolved Quaternary record from the LIB.
Key to investigating the long record preserved in the LIB, obtained by drilling at three sites, is establishment of stratigraphic correlations and a robust chronology. Availability of industry seismic data provides the key to correlate the three planned long sediment cores. Furthermore, workshop participants discussed several suitable chronometric techniques and correlation strategies. We agreed that the LIBRE chronology will combine multiple well-developed techniques. For example, presence of CAVA tephra in outcrops surrounding Lake Izabal (Obrist-Farner et al., 2020) and in the broader region (Kutterolf et al., 2016) suggests that the recovered cores will contain sufficient tephra to establish anchor points for correlations. We will also utilize radiocarbon, optically stimulated luminescence, magnetic stratigraphy, U-series dating, 40Ar 39Ar geochronology, and cosmogenic nuclide dating to establish correlations between core sites and a robust chronology for the LIB records.
Finally, workshop participants discussed the possibility of creating a set of fault observatory boreholes in the vicinity of Lake Izabal (Fig. 7). To establish a borehole-based observatory, drilling in bedrock is necessary to achieve coupling between the cemented borehole and the host rock. Discussions focused on drilling all boreholes in locations where bedrock is exposed at the surface (Fig. 3). All boreholes will be drilled to a depth of 200 m, cased, and instrumented with seismometers and strainmeters. Establishing a borehole-based observatory provides an excellent opportunity to monitor and record earthquake processes in close proximity to active faults. In particular, the borehole observatory will provide rich information for investigating and monitoring microseismicity and strain rates across the system (e.g., Prevedel et al., 2015) and be used to determine fault activity and geometries, which are essential input parameters for subsequent modeling studies that aim to assess the long-term hazards of the plate boundary.
No data sets were used in this article.
The LIBRE Scientific Team: Alejandra Aguilar (University of Notre Dame, USA), Maritza Aguirre (Authority for the Sustainable Management of Lake Izabal, Guatemala), Linus Victor Anyanna (Missouri University of Science and Technology, USA), Cristina Arrieta (Missouri University of Science and Technology, USA), Maria Ask (Lulea University of Technology, Sweden), Rodrigo Barillas (Luis von Ahn Foundation, Guatemala), Thorsten Bauersachs (Christian-Albrechts-Universität, Germany), Melissa Berke (University of Notre Dame, USA), Juan Pablo Bernal (National Autonomous University of Mexico, Mexico), Broxton Bird (Indiana University–Purdue University Indianapolis, USA), Marco Bohnhoff (Helmholtz Centre Potsdam GFZ, Germany), Mark Brenner (University of Florida, USA), Erik Brown (University of Minnesota Duluth, USA), Matthias Buecker (TU Braunschweig, Germany), Caroline Burberry (University of Nebraska–Lincoln, USA), Alex Correa-Metrio (National Autonomous University of Mexico, Mexico), Andrew Cohen (University of Arizona, USA), Jason Curtis (University of Florida, USA), Peter Douglas (McGill University, Canada), Edward Duarte (Savoy Mont Blanc University, France), Paula Echeverría-Galindo (TU Braunschweig, Germany), Andreas Eckert (Missouri University of Science and Technology, USA), Jaime Escobar (Universidad del Norte, Colombia), Alejandro Fernandez (University of Minnesota, USA), Merle Fernandez (National Council for Protected Areas, Guatemala), Omar Flores (San Carlos University, Guatemala), Stephen Gao (Missouri University of Science and Technology, USA), Derek Gibson (Missouri University of Science and Technology, USA), Carla Gordillo (San Carlos University, Guatemala), Christoph Gruetzner (Jena University, Germany), Ulrich Harms (International Continental Scientific Drilling Program, Germany), Robert Hatfield (University of Florida, USA), Carlos Jaramillo (Smithsonian Tropical Research Institute, Panama), Bronwen Konecky (Washington University, St. Louis, USA), Sebastian Krastel (Kiel University, Germany), Stephen Kuehn (Concord University, USA), Steffen Kutterolf (GEOMAR, Germany), Matthew Lachniet (University of Nevada, Las Vegas, USA), Emanuele Lodolo (National Institute of Oceanography and Experimental Geophysics, Italy), Silvia Lopez (National Forest Institute, Guatemala), Julio Luna (San Carlos University, Guatemala), Marta Marchegiano (Vrije University, Belgium), Javier Marquez (NGO Nature Defenders, Guatemala), Patricia Martinez-Garzon (Helmholtz Centre Potsdam GFZ, Germany), Jeremy Maurer (Missouri University of Science and Technology, USA), Trenton McEnaney (Missouri University of Science and Technology, USA), Jasper Moernaut (University of Innsbruck, Austria), Barbara Moguel (National Autonomous University of Mexico, Mexico), Sergio Moran (Northern Central University, Guatemala), Sydney Moser (Missouri University of Science and Technology, USA), Amy Myrbo (Amiable Consulting, USA), Tina Niemi (University of Missouri–Kansas City, USA), Anders Noren (Continental Scientific Drilling Office – University of Minnesota, USA), Francisca Oboh-Ikuenobe (Missouri University of Science and Technology, USA) Jonathan Obrist-Farner (Missouri University of Science and Technology, USA), Bessie Oliva (San Carlos University, Guatemala), Carlos Perez (San Carlos University, Guatemala), Liseth Perez (TU Braunschweig, Germany), Francisco Perez-Sabino (San Carlos University, Guatemala), Brittany Price (Northern Illinois University, USA), Fatima Reyes (Authority for the Sustainable Management of Lake Atitlan, Guatemala), Claudia Romero (University of the Valley of Guatemala, Guatemala), Joshua Rosenfeld (Geological consultant, USA), Daniel Ruiz-Carrascal (Columbia University, USA), Stephanie Scheidt (University of Cologne, Germany), Luca Smeraglia (Italian National Research Council, Italy), Sonia Solis (Luis von Ahn Foundation, Guatemala), Quin Stangeland (University of Minnesota Duluth, USA), Byron Steinman (University of Minnesota Duluth, USA), Jeffery Stone (Indiana State University, USA), Mathias Vinnepand (Leibniz Institute of Applied Geophysics, Germany), Mathew Waters (Auburn University, USA), Nigel Wattrus (University of Minnesota Duluth, USA), Robin Yani (National Institute of Seismology, Volcanology, Meteorology, and Hydrology, Guatemala), Christian Zeeden (Leibniz Institute of Applied Geophysics, Germany), Susan Zimmerman (Lawrence Livermore National Laboratory, USA).
JOF organized the workshop and wrote the first draft of the paper. AE, PMJD, LP, ACM, BLK, TB, SZ, StS, MB, SK, JM, OF, CMB, AN, AM, ML, NW, and DG and the entire LIBRE team co-wrote and edited the final version of the paper and provided intellectual input during the workshop.
The contact author has declared that none of the authors has any competing interests.
Publisher’s note: Copernicus Publications remains neutral with regard to jurisdictional claims in published maps and institutional affiliations.
We are most grateful to Vivian Paniagua from the Camino Real Hotel in Antigua and Ezequiel Insigna from the Nana Juana Hotel in Izabal for help with hotel accommodations and workshop organization. We also thank Maritza Aguirre and Edwin James from the Authority for the Sustainable Management of Lake Izabal for help with organizing the field excursion in Lake Izabal. This work was partially performed under the auspices of the US Department of Energy and by Lawrence Livermore National Laboratory under contract DE-AC52-07NA27344; this is LLNL-JRNL-844029. Finally, we thank editor Nadine Hallman and Bernd Zolitschka and an anonymous reviewer for comments that helped improve the paper.
This research has been supported by the Directorate for Geosciences (grant no. 1939228) and the International Continental Scientific Drilling Program.
This paper was edited by Nadine Hallmann and reviewed by Bernd Zolitschka and one anonymous referee.
Anselmetti, F., Ashwal, L., Ariztegui, D., Bohnhoff, M., Bomberg, M., Claeys, P., Echelberger, J., Ellsworth, W. L., Goodenough, K., Heubeck, C., Kelemen, P., Kipfer, R., Koeberl, C., Kopf, A., Miller, K., Nordgulen, O., Noren, A., Onstott, T., Pease, V., Philippot, P., Russell, J., Soreghan, G., Stein, M., Verschuren, D., and Yamada, Y.: ICDP Science Plan: 2020–2030, International Continental Scientific Drilling Program, Potsdam, https://doi.org/10.2312/icdp.2020.001, 2020.
Auderset, A., Martínez-García, A., Tiedemann, R., Hasenfratz, A. P., Eglinton, T. I., Schiebel, R., Sigman, D. M., and Haug, G. H.: Gulf Stream intensification after the early Pliocene shoaling of the Central American Seaway, Earth Planet. Sc. Lett., 520, 268–278, https://doi.org/10.1016/j.epsl.2019.05.022, 2019.
Authemayou, C., Brocard, G., Teyssier, C., Suski, B., Cosenza, B., Morán-Ical, S., González-Véliz, C. W., Aguilar-Hengstenberg, M. A., and Holliger, K.: Quaternary seismo-tectonic activity of the Polochic Fault, Guatemala, J. Geophys. Res.-Sol. Ea., 117, B07403, https://doi.org/10.1029/2012JB009444, 2012.
Bacon, C. D., Silvestro, D., Jaramillo, C., Smith, B. T., Chakrabarty, P., and Antonelli, A.: Biological evidence supports an early and complex emergence of the Isthmus of Panama, P. Natl. Acad. Sci. USA, 112, 6110, https://doi.org/10.1073/pnas.1423853112, 2015.
Bacon, C. D., Molnar, P., Antonelli, A., Crawford, A. J., Montes, C., and Vallejo-Pareja, M. C.: Quaternary glaciation and the Great American Biotic Interchange, Geology, 44, 375–378, https://doi.org/10.1130/G37624.1, 2016.
Baldwin, J. W., Atwood, A. R., Vecchi, G. A., and Battisti, D. S.: Outsize influence of Central American orography on global climate, AGU Advances, 2, e2020AV000343, https://doi.org/10.1029/2020AV000343, 2021.
Bartole, R., Lodolo, E., Obrist-Farner, J., and Morelli, D.: Sedimentary architecture, structural setting, and Late Cenozoic depocentre migration of an asymmetric transtensional basin: Lake Izabal, eastern Guatemala, Tectonophysics, 750, 419–433, https://doi.org/10.1016/j.tecto.2018.12.004, 2019.
Beeson, J. W., Johnson, S. Y., and Goldfinger, C.: The transtensional offshore portion of the northern San Andreas fault: Fault zone geometry, late Pleistocene to Holocene sediment deposition, shallow deformation patterns, and asymmetric basin growth, Geosphere, 13, 1173–1206, https://doi.org/10.1130/GES01367.1, 2017.
Ben-Avraham, Z. and Zoback, M. D.: Transform-normal extension and asymmetric basins: An alternative to pull-apart models, Geology, 20, 423–426, https://doi.org/10.1130/0091-7613(1992)020<0423:TNEAAB>2.3.CO;2, 1992.
Ben-Zion, Y., Beroza, G. C., Bohnhoff, M., Gabriel, A. A., and Mai, P. M.: A grand challenge international infrastructure for earthquake science, Seismol. Res. Lett., 93, 2967–2968, https://doi.org/10.1785/0220220266, 2022.
Bonis, S., Bohnenberger, O. H., and Dengo, G.: Mapa geológico de la República de Guatemala, Instituto Geográfico Nacional, 1970.
Brierley, C. M. and Fedorov, A. V.: Comparing the impacts of Miocene–Pliocene changes in inter-ocean gateways on climate: Central American Seaway, Bering Strait, and Indonesia, Earth Planet. Sc. Lett., 444, 116–130, https://doi.org/10.1016/j.epsl.2016.03.010, 2016.
Brinson, M. M. and Nordlie, F. G.: II. Lakes. 8. Central and South America: Lake Izabal, Guatemala, SIL Proceedings, 1922–2010, 19, 1468–1479, https://doi.org/10.1080/03680770.1974.11896206, 1975.
Burke, K. D., Williams, J. W., Chandler, M. A., Haywood, A. M., Lunt, D. J., and Otto-Bliesner, B. L.: Pliocene and Eocene provide best analogs for near-future climates, P. Natl. Acad. Sci. USA, 115, 13288–13293, https://doi.org/10.1073/pnas.1809600115, 2018.
Chalk, T. B., Hain, M. P., Foster, G. L., Rohling, E. J., Sexton, P. F., Badger, M. P. S., Cherry, S. G., Hasenfratz, A. P., Haug, G. H., Jaccard, S. L., Martínez-García, A., Pälike, H., Pancost, R. D., and Wilson, P. A.: Causes of ice age intensification across the Mid-Pleistocene Transition, P. Natl. Acad. Sci. USA, 114, 13114–13119, https://doi.org/10.1073/pnas.1702143114, 2017.
Colman, S. M.: Continental drilling and paleoclimate records: Recommendations from an international workshop, PAGES Workshop Report Series, GeoForschungsZentrum, Postdam, 30 June–2 July 1995, Workshop Report, Series 96-4, https://pastglobalchanges.org/sites/default/files/2022-12/1996_Continental_Drilling_PAGES_4_0.pdf (last access: 20 March 2023), 1996.
Correa-Metrio, A., Bush, M. B., Pérez, L., Schwalb, A., and Cabrera, K. R.: Pollen distribution along climatic and biogeographic gradients in northern Central America, Holocene, 21, 681–692, https://doi.org/10.1177/0959683610391321, 2011.
Correa-Metrio, A., Bush, M. B., Cabrera, K. R., Sully, S., Brenner, M., Hodell, D. A., Escobar, J., and Guilderson, T.: Rapid climate change and no-analog vegetation in lowland Central America during the last 86 000 years, Quaternary Sci. Rev., 38, 63–75, https://doi.org/10.1016/j.quascirev.2012.01.025, 2012.
Crowell, J. C.: Introduction to geology of Ridge Basin, southern California, in: Evolution of Ridge Basin, southern California: an interplay of sedimentation and tectonics, edited by: Crowell, J. C., Geological Society of America Special Paper 367, 1–15, Boulder, Colorado, https://doi.org/10.1130/0-8137-2367-1.1, 2003.
D'Hondt, S., Jørgensen, B. B., Miller, D. J., Batzke, A., Blake, R., Cragg, B. A., Cypionka, H., Dickens, G. R., Ferdelman, T., Hinrichs, K.-U., Holm, N. G., Mitterer, R., Spivack, A., Wang, G., Bekins, B., Engelen, B., Ford, K., Gettemy, G., Rutherford, S. D., Sass, H., Skilbeck, C. G., Aiello, I. W., Guèrin, G., House, C. H., Inagaki, F., Meister, P., Naehr, T., Niitsuma, S., Parkes, R. J., Schippers, A., Smith, D. C., Teske, A., Wiegel, J., Padilla, C. N., and Acosta, J. L. S.: Distributions of microbial activities in deep subseafloor sediments, Science, 306, 2216–2221, https://doi.org/10.1126/science.1101155, 2004.
Drexler, J. W., Rose, W. I., Sparks, R. S. J., and Ledbetter, M. T.: The Los Chocoyos Ash, Guatemala: A major stratigraphic marker in Middle America and in three ocean basins, Quaternary Res., 13, 327–345, https://doi.org/10.1016/0033-5894(80)90061-7, 1980.
Duarte, E., Obrist-Farner, J., Correa-Metrio, A., and Steinman, B. A.: A progressively wetter early through middle Holocene climate in the eastern lowlands of Guatemala, Earth Planet. Sc. Lett., 561, 116807, https://doi.org/10.1016/j.epsl.2021.116807, 2021.
Ellis, A., DeMets, C., McCaffrey, R., Briole, P., Cosenza Muralles, B., Flores, O., Guzmán-Speziale, M., Hernández, D., Kostoglodov, V., LaFemina, P., Lord, N., Lasserre, C., Lyon-Caen, H., Rodriguez Maradiaga, M., Molina, E., Rivera, J., Rogers, R., Staller, A., and Tikoff, B.: GPS constraints on deformation in northern Central America from 1999 to 2017, Part 2: Block rotations and fault slip rates, fault locking and distributed deformation, Geophys. J. Int., 218, 729–754, https://doi.org/10.1093/gji/ggz173, 2019.
Feakins, S. J., Liddy, H. M., Tauxe, L., Galy, V., Tierney, J. E., Miao, Y., and Warny, S.: Miocene C4 grassland expansion as recorded by the Indus Fan, Paleoceanography and Paleoclimatology, 35, e2020PA003856, https://doi.org/10.1029/2020PA003856, 2020.
Fedorov, A. V., Brierley, C. M., Lawrence, K. T., Liu, Z., Dekens, P. S., and Ravelo, A. C.: Patterns and mechanisms of early Pliocene warmth, Nature, 496, 43–49, https://doi.org/10.1038/nature12003, 2013.
Fedorov, A. V., Burls, N. J., Lawrence, K. T., and Peterson, L. C.: Tightly linked zonal and meridional sea surface temperature gradients over the past five million years, Nat. Geosci., 8, 975–980, https://doi.org/10.1038/ngeo2577, 2015.
Graham, A.: Studies in Neotropical paleobotany. XI. Late Tertiary vegetation and environments of southeastern Guatemala: palynofloras from the Mio-Pliocene Padre Miguel Group and the Pliocene Herrería Formation, Am. J. Bot., 85, 1409–1425, https://doi.org/10.2307/2446399, 1998.
Graham, A.: Late Cretaceous and Cenozoic history of Latin American vegetation and terrestrial environments, Missouri Botanical Garden Press, ISBN 9781930723689, 2010.
Guzmán-Speziale, M. and Molina, E.: Seismicity and seismically active faulting of Guatemala: A review, J. S. Am. Earth Sci., 115, 103740, https://doi.org/10.1016/j.jsames.2022.103740, 2022.
Harms, U., Koeberl, C., and Zoback, M. D.: Continental scientific drilling: A decade of progress, and challenges for the future, Springer, https://doi.org/10.1007/978-3-540-68778-8, 2007.
Haug, G. H. and Tiedemann, R.: Effect of the formation of the Isthmus of Panama on Atlantic Ocean thermohaline circulation, Nature, 393, 673–676, https://doi.org/10.1038/31447, 1998.
Haug, G. H., Tiedemann, R., Zahn, R., and Ravelo, A. C.: Role of Panama uplift on oceanic freshwater balance, Geology, 29, 207–210, https://doi.org/10.1130/0091-7613(2001)029<0207:ROPUOO>2.0.CO;2, 2001.
Hernández, E., Obrist-Farner, J., Brenner, M., Kenney, W. F., Curtis, J. H., and Duarte, E.: Natural and anthropogenic sources of lead, zinc, and nickel in sediments of Lake Izabal, Guatemala, J. Environ. Sci., 96, 117–126, https://doi.org/10.1016/j.jes.2020.04.020, 2020.
Heuer, V. B., Inagaki, F., Morono, Y., Kubo, Y., Spivack, A. J., Viehweger, B., Treude, T., Beulig, F., Schubotz, F., Tonai, S., Bowden, S. A., Cramm, M., Henkel, S., Hirose, T., Homola, K., Hoshino, T., Ijiri, A., Imachi, H., Kamiya, N., Kaneko, M., Lagostina, L., Manners, H., McClelland, H.-L., Metcalfe, K., Okutsu, N., Pan, D., Raudsepp, M. J., Sauvage, J., Tsang, M.-Y., Wang, D. T., Whitaker, E., Yamamoto, Y., Yang, K., Maeda, L., Adhikari, R. R., Glombitza, C., Hamada, Y., Kallmeyer, J., Wendt, J., Wörmer, L., Yamada, Y., Kinoshita, M., and Hinrichs, K.-U.: Temperature limits to deep subseafloor life in the Nankai Trough subduction zone, Science, 370, 1230–1234, https://doi.org/10.1126/science.abd7934, 2020.
Holt, B. G., Lessard, J.-P., Borregaard, M. K., Fritz, S. A., Araújo, M. B., Dimitrov, D., Fabre, P.-H., Graham, C. H., Graves, G. R., Jønsson, K. A., Nogués-Bravo, D., Wang, Z., Whittaker, R. J., Fjeldså, J., and Rahbek, C.: An Update of Wallace's zoogeographic regions of the world, Science, 339, 74–78, https://doi.org/10.1126/science.1228282, 2013.
Huntington, K. W. and Klepeis, K. A.: Challenges and opportunities for research in tectonics: Understanding deformation and the processes that link Earth systems, from geologic time to human time. A community vision document submitted to the U.S. National Science Foundation, University of Washington, 84 pp., http://hdl.handle.net/1773/40754 (last access: 17 March 2023), 2018.
Inagaki, F., Hinrichs, K. U., Kubo, Y., Bowles, M. W., Heuer, V. B., Hong, W. L., Hoshino, T., Ijiri, A., Imachi, H., Ito, M., Kaneko, M., Lever, M. A., Lin, Y. S., Methé, B. A., Morita, S., Morono, Y., Tanikawa, W., Bihan, M., Bowden, S. A., Elvert, M., Glombitza, C., Gross, D., Harrington, G. J., Hori, T., Li, K., Limmer, D., Liu, C. H., Murayama, M., Ohkouchi, N., Ono, S., Park, Y. S., Phillips, S. C., Prieto-Mollar, X., Purkey, M., Riedinger, N., Sanada, Y., Sauvage, J., Snyder, G., Susilawati, R., Takano, Y., Tasumi, E., Terada, T., Tomaru, H., Trembath-Reichert, E., Wang, D. T., and Yamada, Y.: Exploring deep microbial life in coal-bearing sediment down to ∼ 2.5 km below the ocean floor, Science, 349, 420–424, https://doi.org/10.1126/science.aaa6882, 2015.
Jaramillo, C.: Evolution of the Isthmus of Panama: biological, paleoceanographic and paleoclimatological implications, in: Mountains, climate and biodiversity, edited by: Hoorn, C., Perrigo, A., and Antonelli, A., Wiley-Blackwell, 323–338, ISBN 978-1-119-15988-9, 2018.
Jaramillo, C., Sepulchre, P., Cardenas, D., Correa-Metrio, A., Moreno, J. E., Trejos, R., Vallejos, D., Hoyos, N., Martínez, C., Carvalho, D., Escobar, J., Oboh-Ikuenobe, F., Prámparo, M. B., and Pinzón, D.: Drastic vegetation change in the Guajira Peninsula (Colombia) during the Neogene, Paleoceanography and Paleoclimatology, 35, e2020PA003933, https://doi.org/10.1029/2020PA003933, 2020.
Jordan, B. R., Sigurdsson, H., Carey, S., Lundin, S., Rogers, R. D., Singer, B., and Barquero-Molina, M.: Petrogenesis of Central American Tertiary ignimbrites and associated Caribbean Sea tephra, in: Geologic and Tectonic Development of the Caribbean Plate Boundary in Northern Central America, edited by: Mann, P., Geological Society of America, https://doi.org/10.1130/2007.2428(07), 2007.
Kallmeyer, J., Pockalny, R., Adhikari, R. R., Smith, D. C., and D'Hondt, S.: Global distribution of microbial abundance and biomass in subseafloor sediment, P. Natl. Acad. Sci. USA, 109, 16213–16216, https://doi.org/10.1073/pnas.1203849109, 2012.
Kutterolf, S., Freundt, A., and Peréz, W.: Pacific offshore record of Plinian arc volcanism in Central America: 2. Tephra volumes and erupted masses, Geochem. Geophy. Geosy., 9, Q02S02, https://doi.org/10.1029/2007GC001791, 2008a.
Kutterolf, S., Freundt, A., Peréz, W., Mörz, T., Schacht, U., Wehrmann, H., and Schmincke, H. U.: Pacific offshore record of Plinian arc volcanism in Central America: 1. Along-arc correlations, Geochem. Geophy. Geosy., 9, Q02S01, https://doi.org/10.1029/2007GC001631, 2008b.
Kutterolf, S., Schindlbeck, J. C., Anselmetti, F. S., Ariztegui, D., Brenner, M., Curtis, J., Schmid, D., Hodell, D. A., Mueller, A., Pérez, L., Pérez, W., Schwalb, A., Frische, M., and Wang, K. L.: A 400-ka tephrochronological framework for Central America from Lake Petén Itzá (Guatemala) sediments, Quaternary Sci. Rev., 150, 200–220, https://doi.org/10.1016/j.quascirev.2016.08.023, 2016.
Lodolo, E., Menichetti, M., Guzmán-Speziale, M., Giunta, G., and Zanolla, C.: Deep structural setting of the North American-Caribbean plate boundary in eastern Guatemala, Geofís. Int., 48, 263–277, 2009.
Lyon-Caen, H., Barrier, E., Lasserre, C., Franco, A., Arzu, I., Chiquin, L., Chiquin, M., Duquesnoy, T., Flores, O., Galicia, O., Luna, J., Molina, E., Porras, O., Requena, J., Robles, V., Romero, J., and Wolf, R.: Kinematics of the North American–Caribbean-Cocos plates in Central America from new GPS measurements across the Polochic-Motagua fault system, Geophys. Res. Lett., 33, L19309, https://doi.org/10.1029/2006GL027694, 2006.
Mann, P. (Ed.): Overview of the tectonic history of northern Central America, in: Geologic and tectonic development of the Caribbean plate boundary in northern Central America, Geological Society of America Special Paper 428, 1–19, https://doi.org/10.1130/2007.2428(01), 2007.
McGirr, R., Seton, M., and Williams, S.: Kinematic and geodynamic evolution of the Isthmus of Panama region: Implications for Central American Seaway closure, GSA Bulletin, 133, 867–884, https://doi.org/10.1130/B35595.1, 2020.
Molnar, P.: Closing of the Central American Seaway and the Ice Age: A critical review, Paleoceanography, 23, PA2201, https://doi.org/10.1029/2007PA001574, 2008.
Mongol, E., Oboh-Ikuenobe, F., Obrist-Farner, J., Moreno, J. E., and Correa-Metrio, A.: A millennium of anthropic and climate dynamics in the Lake Izabal Basin, eastern lowland Guatemala, Rev. Palaeobot. Palyno., 312, 104872, https://doi.org/10.1016/j.revpalbo.2023.104872, 2023.
Mota-Vidaure, A. B.: Stratigraphy of the coal-bearing strata (Miocene) in the Carboneras Region, Izabal, Guatemala, Department of Geology and Geological Engineering, Colorado School of Mines, 104 pp., https://hdl.handle.net/11124/172218 (last access: 17 March 2023), 1989.
NASEM: National Academies of Sciences, E., and Medicine: A vision for NSF Earth Sciences 2020–2030: Earth in Time, The National Academies Press, https://doi.org/10.17226/25761, 2020.
National Research Council (NRC): Scientific Ocean drilling: Accomplishments and challenges, The National Academies Press, Washington, DC, 158 pp., https://doi.org/10.17226/13232, 2011.
Obrist-Farner, J., Brenner, M., Curtis, J. H., Kenney, W. F., and Salvinelli, C.: Recent onset of eutrophication in Lake Izabal, the largest water body in Guatemala, J. Paleolimnol., 62, 359–372, https://doi.org/10.1007/s10933-019-00091-3, 2019.
Obrist-Farner, J., Eckert, A., Locmelis, M., Crowley, J. L., Mota-Vidaure, B., Lodolo, E., Rosenfeld, J., and Duarte, E.: The role of the Polochic Fault as part of the North American and Caribbean Plate boundary: Insights from the infill of the Lake Izabal Basin, Basin Res., 32, 1347–1364, https://doi.org/10.1111/bre.12431, 2020.
Obrist-Farner, J., Brenner, M., Stone, J. R., Wojewódka-Przybył, M., Bauersachs, T., Eckert, A., Locmelis, M., Curtis, J. H., Zimmerman, S. R. H., Correa-Metrio, A., Schwark, L., Duarte, E., Schwalb, A., Niewerth, E., Echeverría-Galindo, P. G., and Pérez, L.: New estimates of the magnitude of the sea-level jump during the 8.2 ka event, Geology, 50, 86–90, https://doi.org/10.1130/G49296.1, 2022.
Obrist-Farner, J., Steinman, B. A., Stansell, N. D., and Maurer, J.: Incoherency in Central American hydroclimate proxy records spanning the last millennium, Paleoceanography and Paleoclimatology, 38, e2022PA004445, https://doi.org/10.1029/2022PA004445, 2023.
O'Dea, A., Lessios, H. A., Coates, A. G., Eytan, R. I., Restrepo-Moreno, S. A., Cione, A. L., Collins, L. S., de Queiroz, A., Farris, D. W., Norris, R. D., Stallard, R. F., Woodburne, M. O., Aguilera, O., Aubry, M.-P., Berggren, W. A., Budd, A. F., Cozzuol, M. A., Coppard, S. E., Duque-Caro, H., Finnegan, S., Gasparini, G. M., Grossman, E. L., Johnson, K. G., Keigwin, L. D., Knowlton, N., Leigh, E. G., Leonard-Pingel, J. S., Marko, P. B., Pyenson, N. D., Rachello-Dolmen, P. G., Soibelzon, E., Soibelzon, L., Todd, J. A., Vermeij, G. J., and Jackson, J. B. C.: Formation of the Isthmus of Panama, Sci. Adv., 2, e1600883, https://doi.org/10.1126/sciadv.1600883, 2016.
Pagani, M., Freeman, K. H., and Arthur, M. A.: Late Miocene atmospheric CO2 concentrations and the expansion of C4 grasses, Science, 285, 876–879, https://doi.org/10.1126/science.285.5429.876, 1999.
Parkes, R. J., Cragg, B. A., Bale, S. J., Getlifff, J. M., Goodman, K., Rochelle, P. A., Fry, J. C., Weightman, A. J., and Harvey, S. M.: Deep bacterial biosphere in Pacific Ocean sediments, Nature, 371, 410–413, https://doi.org/10.1038/371410a0, 1994.
Pérez, L., Correa-Metrio, A., Cohuo, S., González, L. M., Echeverría-Galindo, P., Brenner, M., Curtis, J., Kutterolf, S., Stockhecke, M., Schenk, F., Bauersachs, T., and Schwalb, A.: Ecological turnover in neotropical freshwater and terrestrial communities during episodes of abrupt climate change, Quaternary Res., 101, 26–36, https://doi.org/10.1017/qua.2020.124, 2021.
Plafker, G.: Tectonic aspects of the Guatemala earthquake of 4 February 1976, Science, 193, 1201–1208, https://doi.org/10.1126/science.193.4259.1201, 1976.
Powers, S.: Notes on the geology of eastern Guatemala and northwestern Spanish Honduras, J. Geol., 26, 507–523, 1918.
Prance, G. T.: A review of the phytogeographic evidences for Pleistocene climate changes in the Neotropics, An. MO Bot. Gard., 69, 594–624, https://doi.org/10.2307/2399085, 1982.
Prevedel, B., Bulut, F., Bohnhoff, M., Raub, C., Kartal, R. F., Alver, F., and Malin, P. E.: Downhole geophysical observatories: best installation practices and a case history from Turkey, Int. J. Earth Sci., 104, 1537–1547, https://doi.org/10.1007/s00531-015-1147-5, 2015.
Salzmann, U., Haywood, A. M., Lunt, D. J., Valdes, P. J., and Hill, D. J.: A new global biome reconstruction and data-model comparison for the Middle Pliocene, Global Ecol. Biogeogr., 17, 432–447, https://doi.org/10.1111/j.1466-8238.2008.00381.x, 2008.
Schindlbeck, J. C., Kutterolf, S., Freundt, A., Eisele, S., Wang, K. L., and Frische, M.: Miocene to Holocene marine tephrostratigraphy offshore northern Central America and southern Mexico: Pulsed activity of known volcanic complexes, Geochem. Geophy. Geosy., 19, 4143–4173, https://doi.org/10.1029/2018GC007832, 2018.
Sentman, L. T., Dunne, J. P., Stouffer, R. J., Krasting, J. P., Toggweiler, J. R., and Broccoli, A. J.: The mechanistic role of the Central American seaway in a GFDL Earth System model. Part 1: Impacts on global ocean mean state and circulation, Paleoceanography and Paleoclimatology, 33, 840–859, https://doi.org/10.1029/2018PA003364, 2018.
Sigurdsson, H., Kelley, S., Leckie, R. M., Carey, S., Bralower, T., and King, J.: History of circum-Caribbean explosive volcanism: 40Ar 39Ar dating of tephra layers, in: Proceedings of the Ocean Drilling Program, Scientific Results, edited by: Leckie, R. M., Sigurdsson, H., Acton, G. D., and Draper, G., 299–314, https://doi.org/10.2973/odp.proc.sr.165.021.2000, 2000.
Soreghan, G. S. and Cohen, A. S.: Scientific drilling and the evolution of the earth system: climate, biota, biogeochemistry and extreme systems, Sci. Dril., 16, 63–72, https://doi.org/10.5194/sd-16-63-2013, 2013.
Strömberg, C. A. E.: Evolution of grasses and grassland ecosystems, Annu. Rev. Earth Pl. Sc., 39, 517–544, https://doi.org/10.1146/annurev-earth-040809-152402, 2011.
Strömberg, C. A. E. and McInerney, F. A.: The Neogene transition from C3 to C4 grasslands in North America: assemblage analysis of fossil phytoliths, Paleobiology, 37, 50–71, https://doi.org/10.1666/09067.1, 2011.
Tsai, V. C., Hirth, G., Trugman, D. T., and Chu, S. X.: Impact versus fractional earthquake models for high-frequency radiation in complex fault zones, J. Geophys. Res.-Sol. Ea., 126, e2021JB022313, https://doi.org/10.1029/2021JB022313, 2021.
Uno, K. T., Cerling, T. E., Harris, J. M., Kunimatsu, Y., Leakey, M. G., Nakatsukasa, M., and Nakaya, H.: Late Miocene to Pliocene carbon isotope record of differential diet change among East African herbivores, P. Natl. Acad. Sci. USA, 108, 6509–6514, https://doi.org/10.1073/pnas.1018435108, 2011.
Utescher, T., Dreist, A., Henrot, A.-J., Hickler, T., Liu, Y.-S., Mosbrugger, V., Portmann, F. T., and Salzmann, U.: Continental climate gradients in North America and Western Eurasia before and after the closure of the Central American Seaway, Earth Planet. Sc. Lett., 472, 120–130, https://doi.org/10.1016/j.epsl.2017.05.019, 2017.
Vaughan, T. W.: The biologic character and geological correlation of the sedimentary formations of Panama in their relation to the geologic history of Central America and the West Indies, U.S. National Museum Bulletin, 103, 547–612, 1919.
Vinson, G. L.: Upper Cretaceous and Tertiary stratigraphy of Guatemala, AAPG Bull., 46, 425–456, https://doi.org/10.1306/BC743835-16BE-11D7-8645000102C1865D, 1962.
White, R. A.: Catalog of historic seismicity in the vicinity of the Chixoy-Polochic and Motagua faults, Guatemala, USGS Report 84-88, https://doi.org/10.3133/ofr8488, 1984.
White, R. A.: The Guatemala earthquake of 1816 on the Chixoy-Polochic fault, B. Seismol. Soc. Am., 75, 455–473, 1985.
- Abstract
- Introduction
- The Lake Izabal Basin Research Endeavor (LIBRE) project
- Current knowledge of the Lake Izabal Basin
- The Lake Izabal Basin – a world-class scientific drilling site
- The LIBRE scientific drilling plan and site selection
- Data availability
- Team list
- Author contributions
- Competing interests
- Disclaimer
- Acknowledgements
- Financial support
- Review statement
- References
- Abstract
- Introduction
- The Lake Izabal Basin Research Endeavor (LIBRE) project
- Current knowledge of the Lake Izabal Basin
- The Lake Izabal Basin – a world-class scientific drilling site
- The LIBRE scientific drilling plan and site selection
- Data availability
- Team list
- Author contributions
- Competing interests
- Disclaimer
- Acknowledgements
- Financial support
- Review statement
- References